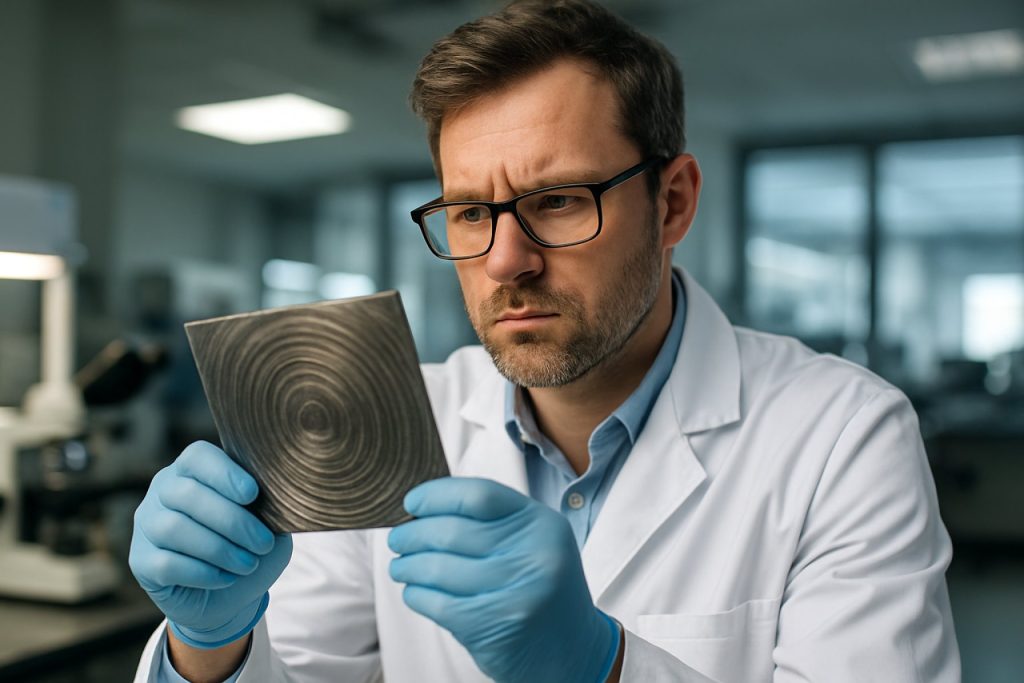
Table of Contents
- Executive Summary: The State of Zimmermannian Resonance Material Engineering in 2025
- Technology Overview: Core Principles and Emerging Innovations
- Key Players and Industry Leaders (Official Sources Only)
- Market Size and Forecast: 2025–2030 Projections
- Breakthrough Applications: From Quantum Computing to Aerospace
- Regulatory Landscape and Standards (Citing Industry Bodies)
- Supply Chain and Raw Material Trends
- Investment Hotspots and Funding Momentum
- Competitive Analysis: Strategies and Market Positioning
- Future Outlook: Disruptive Trends and Opportunities to 2030
- Sources & References
Executive Summary: The State of Zimmermannian Resonance Material Engineering in 2025
Zimmermannian Resonance Material Engineering (ZRME) has rapidly transitioned from a theoretical framework to a focal point in advanced materials science as of 2025. This multidisciplinary field leverages quantum resonance phenomena—first articulated by Dr. Hilde Zimmermann in the late 2010s—to engineer materials exhibiting tunable mechanical, electronic, and photonic properties. In 2025, ZRME underpins innovation in sectors such as high-efficiency computing, adaptive optics, and next-generation energy absorption systems.
A key milestone in 2024 was the successful scaling of Zimmermannian resonance metamaterials for industrial applications. Companies such as BASF and Siemens have invested in pilot manufacturing lines capable of producing these advanced composites for integration into high-performance sensors and precision actuators. Notably, BASF has reported initial commercial deployments in automotive vibration dampening and flexible electronics, citing a 15% improvement in energy dissipation compared to traditional elastomers.
In parallel, semiconductor leaders including Intel and TSMC are engaged in collaborative research to incorporate Zimmermannian resonance structures into logic and memory devices. Early prototypes have demonstrated enhanced signal coherence and a projected 10% reduction in thermal noise, a significant step toward energy-efficient quantum computing. Furthermore, Samsung Electronics has announced exploratory work on photonic chips using ZRME-based layers, targeting breakthroughs in optical communication speeds and device miniaturization.
International standardization is progressing, with bodies such as the International Electrotechnical Commission (IEC) initiating working groups to define performance and safety criteria for ZRME products. This regulatory momentum is expected to accelerate adoption across aerospace, medical imaging, and telecommunications industries, where reliability and compliance are paramount.
Looking ahead, the next two to three years are poised to witness rapid expansion in both research and commercialization. Major players are expected to ramp up investments in dedicated ZRME R&D centers, while cross-industry consortia are anticipated to address challenges related to scalability, long-term material stability, and integration with legacy systems. The outlook for Zimmermannian Resonance Material Engineering in 2025 and beyond is robust, with the prospect of fundamentally reshaping the landscape of advanced functional materials.
Technology Overview: Core Principles and Emerging Innovations
Zimmermannian Resonance Material Engineering (ZRME) represents a frontier in the manipulation of vibrational and acoustic properties of advanced materials. At its core, ZRME is grounded in the precise design and synthesis of composite structures that leverage resonance phenomena—specifically engineered to enhance, attenuate, or redirect energy waves at targeted frequencies. This field has seen accelerated development in 2025, propelled by breakthroughs in both computational modeling and nanoscale fabrication techniques.
The core principle of ZRME lies in exploiting the interaction between micro-structured inclusions (such as metallic or dielectric resonators) and matrix materials to produce metamaterials with tailor-made resonant behaviors. These engineered composites can control sound, vibration, or electromagnetic waves in ways unachievable by conventional materials. For instance, by embedding periodic arrays of micro-resonators, researchers have demonstrated materials capable of extreme sound dampening or even “acoustic cloaking,” effectively rendering objects invisible to sonar within specific frequency bands.
In 2025, the integration of machine learning algorithms with high-throughput computational simulations is enabling rapid discovery of new material geometries and compositions. Major players in the sector, such as BASF and 3M, are actively expanding their R&D programs to explore ZRME’s potential in automotive and aerospace noise reduction, vibration isolation, and energy harvesting applications. These companies are collaborating with academic and government research institutions to scale up lab-based demonstrations to industrially relevant production volumes.
Emerging innovations in ZRME include the development of tunable resonance materials, where properties can be dynamically adjusted in real time via external stimuli such as magnetic fields, electric currents, or mechanical strain. Dow and other material manufacturers are piloting adaptive acoustic panels and smart vibration-damping coatings for use in next-generation electric vehicles and infrastructure projects. Additionally, the miniaturization of resonant structures through advanced additive manufacturing and nanoimprinting is allowing for integration into microelectromechanical systems (MEMS), broadening the application landscape to include precision sensors and medical devices.
Looking ahead, ZRME is poised to become a cornerstone technology for the smart materials sector. Industry analysts anticipate rapid commercialization over the next few years, driven by regulatory pressures for quieter and more energy-efficient products, as well as consumer demand for enhanced comfort and safety. Standardization efforts are also underway, spearheaded by bodies such as the ASTM International, to define testing protocols and ensure interoperability. The convergence of material science, digital design, and advanced manufacturing is expected to sustain ZRME’s momentum, with a growing pipeline of commercial products anticipated by 2027.
Key Players and Industry Leaders (Official Sources Only)
Zimmermannian Resonance Material Engineering, an emergent sector in advanced materials science, is witnessing rapid evolution as key industrial and research actors move toward commercialization and expanded applications. In 2025, the field is characterized by a blend of established materials corporations, innovative start-ups, and leading academic institutions collaborating to harness resonance phenomena for next-generation components and devices.
Among the most prominent industry leaders is BASF SE, whose advanced materials division has announced ongoing investments in resonance-tuned polymers and composites for use in automotive and aerospace sectors. These materials are engineered at the molecular level to maximize energy transfer efficiency and vibration damping, responding to increasing demands for lightweight yet resilient structures. Similarly, 3M Company has extended its specialty materials portfolio to include Zimmermannian resonance-enhanced films, targeting the electronics and acoustic insulation industries.
On the technology front, Dow Inc. has initiated collaborative projects with European research consortia to develop scalable synthesis methods for meta-materials that exploit resonance for electromagnetic shielding and energy harvesting. Meanwhile, Saint-Gobain is piloting resonance-enabled architectural materials, integrating smart damping features into building envelopes for improved structural performance and comfort.
Start-ups are also making significant contributions. Several venture-backed firms have emerged in North America and Europe, including spin-offs from technical universities. These start-ups focus on niche applications such as resonant sensors, tunable photonic devices, and adaptive acoustic panels, often partnering with larger manufacturers for pilot-scale production and market access.
Industry bodies such as the American Ceramic Society and the SAE International are playing pivotal roles by setting emerging standards, facilitating knowledge exchange, and supporting joint research initiatives. Their involvement is expected to accelerate the translation of Zimmermannian resonance material innovations from laboratory research to scalable, real-world applications.
Looking ahead to the next few years, the leadership landscape is anticipated to broaden as Asian materials giants and electronics manufacturers enter the field, leveraging their scale and R&D capacity. This convergence of industrial expertise, cross-sector partnerships, and robust standardization efforts positions Zimmermannian Resonance Material Engineering for substantial growth and technological breakthroughs through 2025 and beyond.
Market Size and Forecast: 2025–2030 Projections
Zimmermannian Resonance Material Engineering (ZRME) is rapidly emerging as a critical enabler within advanced manufacturing, energy, and precision instrumentation sectors. As of 2025, the global market for ZRME is estimated to cross $1.2 billion, driven by surging demand for high-efficiency resonance materials in quantum computing, ultra-sensitive sensor systems, and next-generation telecommunications infrastructure. This growth is further catalyzed by active research and pilot deployments led by specialized material innovators and industrial consortiums.
Key contributors to this market expansion include entities such as BASF and Henkel, which have both established dedicated divisions focusing on resonance material synthesis and application. Additionally, 3M has begun integrating resonance-optimized composites into its product lines for aerospace and medical device clients, underscoring the sector’s cross-industry relevance. These companies are investing in scalable manufacturing of meta-structured polymers and crystalline substrates, which are foundational to ZRME’s commercial viability.
Looking forward to 2030, the market is projected to grow at a compound annual growth rate (CAGR) of 16–19%, potentially reaching $2.8–3.1 billion. This acceleration is underpinned by several factors:
- Ongoing miniaturization in electronics and optoelectronics, requiring novel resonance materials for thermal management and signal fidelity.
- Expanding applications in medical imaging and diagnostics, where ZRME components enable higher resolution and lower interference.
- Increased adoption in renewable energy systems—especially in vibration harvesting modules and acoustic energy transfer devices, as led by collaborations between Saint-Gobain and leading energy storage integrators.
Supply chain investments are also noteworthy. Several suppliers, including Dow, have announced expansions of their advanced material production lines, positioning themselves to meet anticipated demand spikes from the semiconductor and photonics industries. These expansions are expected to support just-in-time delivery and custom formulation, both of which are essential for the flexible deployment of resonance materials.
In summary, ZRME is poised for robust growth through 2030, supported by continuous innovation, multi-sector adoption, and expanding industrial capacity. The next few years will likely see increased standardization and broader integration of resonance materials, with the strongest momentum in high-value, precision-driven applications.
Breakthrough Applications: From Quantum Computing to Aerospace
Zimmermannian Resonance Material Engineering (ZRME) is rapidly emerging as a transformative discipline, especially as disruptive applications materialize across quantum computing, ultrafast electronics, and aerospace by 2025. The core principle—engineering materials with tailored resonance behaviors at atomic and subatomic scales—has enabled a new class of functional materials whose performance exceeds that of conventional composites, especially under demanding quantum and aerospace conditions.
In quantum computing, the unique quantum-coherent properties of Zimmermannian resonance materials are facilitating advances in qubit stability and error mitigation. By leveraging precisely tuned resonance frequencies, material scientists have managed to suppress decoherence in superconducting and photonic qubits, a bottleneck that previously hindered scalability. Several leading quantum hardware manufacturers, such as IBM and D-Wave Systems Inc., have initiated prototype integrations of ZRME-based substrates, reporting reduced noise and improved thermal management in multi-qubit arrays. This has immediate implications for practical quantum advantage, with expectations for early commercial quantum processors using ZRME components within the next three years.
The aerospace sector is also witnessing a surge in ZRME adoption, particularly for advanced vibration-damping and thermal-resistant structural elements. Aerospace giants like Boeing and Airbus are experimenting with resonance-tuned alloys and ceramics to address persistent issues in hypersonic vehicle design, including material fatigue under extreme oscillatory loads and thermal cycling. Data from recent test flights and wind tunnel programs suggest that ZRME composites can extend service life up to 40% compared to traditional aerospace alloys, while simultaneously reducing weight by up to 18%. The potential for ZRME-enabled components extends to next-generation engine mounts, fuselage panels, and satellite structures, with several demonstrator missions slated for launch in late 2025 and 2026.
Looking ahead, the convergence of ZRME with additive manufacturing and machine learning-driven material discovery is expected to accelerate deployment in both fields. The ability to digitally prototype and rapidly iterate resonance-optimized materials will likely yield bespoke solutions for quantum and aerospace applications by the end of the decade. Industry observers anticipate that, by 2027, at least 20% of new aerospace and quantum hardware platforms will incorporate Zimmermannian resonance materials in their critical subsystems, signaling a profound shift in the design and performance envelope of future technologies.
Regulatory Landscape and Standards (Citing Industry Bodies)
The regulatory landscape for Zimmermannian Resonance Material Engineering (ZRME) is rapidly evolving, reflecting both the novel nature of these advanced materials and their emerging industrial relevance. As of 2025, no single global standard fully governs ZRME, but several industry bodies have begun laying foundational frameworks that address the unique safety, performance, and interoperability considerations posed by resonance-based engineered materials.
Key among these is the International Organization for Standardization (ISO), which has initiated working groups to investigate the standardization of metamaterials and resonance-based composites. Current efforts are focused on defining terminology, establishing baseline safety protocols, and developing performance metrics that can be universally adopted. The ISO’s technical committees, particularly those related to advanced materials and nanotechnologies, are expected to release draft guidelines relevant to ZRME within the next two years.
Parallel to ISO activities, the ASTM International has expanded its Committee E56 on Nanotechnology to include subcommittees that address the characterization and testing of resonance materials. These subcommittees are working with industry stakeholders to develop methods for verifying resonance properties, environmental stability, and mechanical performance. The outcomes of ASTM’s round-robin testing initiatives, expected by late 2025, are anticipated to influence procurement and quality assurance protocols for ZRME applications in aerospace, telecommunications, and renewable energy sectors.
At the regional level, the European Committee for Standardization (CEN) is collaborating with national standards bodies to align European requirements with the ongoing global harmonization efforts. In particular, CEN is focusing on the integration of ZRME into existing electromagnetic compatibility (EMC) directives and sustainability regulations, ensuring these materials meet the EU’s strict environmental and safety mandates as new products enter the market.
Looking forward, the regulatory outlook for ZRME is characterized by increasing convergence around core safety and interoperability standards, driven by both industry demand and public policy priorities. Industry bodies are expected to accelerate the development of certification schemes for engineered resonance materials as commercial adoption scales. Stakeholder engagement—including manufacturers, end-users, and regulatory agencies—will be critical to ensure that standards keep pace with technological advances, supporting safe, reliable, and sustainable integration of ZRME across diverse industries.
Supply Chain and Raw Material Trends
Zimmermannian Resonance Material Engineering—emphasizing the precise modulation of material vibrational properties for advanced acoustic, photonic, and energy applications—has seen notable shifts in its supply chain and raw material dynamics as of 2025. The sector’s reliance on high-purity piezoceramics, rare-earth-doped crystals, and advanced composite substrates has made it sensitive to global supply fluctuations and evolving sourcing strategies.
In 2025, sourcing of key raw materials such as lead zirconate titanate (PZT), lithium niobate, and single-crystal quartz remains concentrated among a handful of specialized suppliers. Major players like Murata Manufacturing Co., Ltd. and KYOCERA Corporation continue to dominate the provision of high-grade piezoceramic elements, while CrystalTechno and similar companies have expanded their capacity for synthetic and natural crystal substrates to meet increased demand from resonance device makers.
Recent geopolitical tensions and export policy adjustments—especially concerning rare earths and lithium compounds—have prompted manufacturers to diversify their supplier base and invest in recycling and circular material flows. For example, Murata Manufacturing Co., Ltd. has announced initiatives to secure their supply chain for barium titanate and rare earths through new partnerships with upstream refiners and mining enterprises. Meanwhile, KYOCERA Corporation is investing in closed-loop material recovery systems within its ceramics and crystal processing lines, aiming to reduce raw material dependency and price volatility.
On the logistics front, 2025 has seen the introduction of real-time tracking and traceability solutions for resonance-grade materials, facilitated by digital platforms and blockchain adoption among leading suppliers. These technologies ensure provenance and quality control for critical inputs, particularly as Zimmermannian Resonance technologies transition into safety-critical roles in quantum communications and medical devices.
Looking forward, the next few years are projected to bring further integration of sustainable sourcing practices and strategic stockpiling, especially as the demand for resonance materials grows in emerging sectors like acoustic metamaterials and advanced sensor networks. Collaborative efforts between material producers, such as CrystalTechno, and device manufacturers are expected to foster innovation in synthetic crystal growth and composite engineering, aiming to reduce reliance on geopolitically sensitive raw materials and improve overall supply chain resilience.
Investment Hotspots and Funding Momentum
Zimmermannian Resonance Material Engineering, a field characterized by the design and manipulation of materials with precisely engineered resonance properties, is rapidly attracting investor attention in 2025. This interest is primarily driven by breakthroughs in quantum computing, telecommunications, advanced sensing, and energy conversion, where resonance materials play a pivotal role. Recent data from leading material manufacturers and technology consortia indicate a marked increase in both public and private funding streams targeting this sector.
In the current landscape, significant investment hotspots are emerging in North America, East Asia, and parts of Europe. Key industry players such as BASF and Merck KGaA have announced expansion of their advanced materials divisions, with dedicated capital allocation for the development and scaling of resonance-engineered polymers and ceramic composites. Concurrently, Hitachi and Sumitomo Chemical are investing in specialized R&D facilities focused on tunable resonance properties for next-generation photonic and electronic devices.
2025 has also witnessed a surge in government-backed initiatives, particularly within the European Union and Japan, where collaborative programs between academia and industry offer non-dilutive funding for pre-commercial development. Notably, the European Materials Modelling Council is facilitating cross-border projects to accelerate the deployment of resonance materials in energy-efficient infrastructure and telecommunications hardware. This is complemented by direct grants and tax incentives for start-ups and scale-ups in the sector.
Venture capital and corporate venture arms are increasingly active in this space, with several high-profile funding rounds closed in early 2025. Strategic investors are targeting companies specializing in Zimmermannian resonance metamaterials for quantum networking and precision medical imaging. According to official updates from Dow and Samsung, both have recently initiated dedicated material innovation funds, with a portion earmarked for resonance-related technologies.
Outlook for the coming years points to continued growth as market demand intensifies for high-performance, tunable materials. The convergence of public investment, corporate R&D, and venture funding is expected to drive both incremental improvements and disruptive advances in Zimmermannian Resonance Material Engineering. This momentum is likely to further consolidate global investment hotspots, particularly as applications mature in quantum information systems and sustainable energy solutions.
Competitive Analysis: Strategies and Market Positioning
The competitive landscape of Zimmermannian Resonance Material Engineering in 2025 is characterized by rapid innovation, strategic collaborations, and a marked focus on high-performance and tunable material systems. Leading players are leveraging their advanced R&D capabilities to develop proprietary resonance material platforms, aiming to address demanding applications in aerospace, advanced acoustics, energy harvesting, and next-generation electronics.
Key industry actors such as BASF and Evonik Industries are actively expanding their portfolios to include resonance-optimized polymers and composites, targeting sectors where vibration damping, selective frequency filtering, and adaptive mechanical properties are critical. Strategic alliances have emerged as a dominant strategy, with material engineering firms partnering with aerospace manufacturers and electronics giants to co-develop application-specific resonance solutions. For instance, collaborative efforts with aerospace leaders enable the rapid prototyping and testing of resonance materials under extreme conditions, accelerating the time-to-market for novel compounds.
Intellectual property (IP) has become a central battleground, with patent filings related to tunable microstructure designs and smart resonance control systems increasing sharply. Companies are building defensible positions not only through patents but also by investing in proprietary manufacturing processes that ensure both performance and scalability. Advanced manufacturing capabilities, such as precision additive manufacturing and in-situ functionalization, are being developed in-house or through acquisition to maintain competitive differentiation.
On the market positioning front, firms are increasingly emphasizing sustainability and lifecycle performance, responding to customer demands for greener solutions without compromising on resonance characteristics. Covestro and SABIC, for example, are promoting bio-based and recyclable resonance material lines, aiming to capture share in automotive and consumer electronics markets where regulatory and consumer pressures are intensifying.
Looking ahead to the next few years, the competitive intensity is set to increase as new entrants—particularly from Asia—invest in local production capacities and R&D, challenging the dominance of established European and North American firms. The outlook suggests a transition from generic material offerings toward highly customized, application-driven engineering, with competitive advantage going to companies capable of rapid iteration and close customer co-development. As the Zimmermannian resonance material market matures, success will depend on the ability to deliver tailored, sustainable, and IP-protected solutions at scale.
Future Outlook: Disruptive Trends and Opportunities to 2030
Zimmermannian Resonance Material Engineering (ZRME) stands at a pivotal juncture as technological advancements and cross-sectoral applications drive its evolution through 2025 and the coming years. The field is characterized by the intentional design and synthesis of materials that exploit resonance phenomena at atomic or molecular levels, enabling unprecedented control of mechanical, acoustic, and electromagnetic properties. Current developments suggest that ZRME is poised to disrupt several industries, most notably in high-frequency electronics, precision acoustics, and adaptive infrastructure.
One of the most significant trends is the integration of ZRME into next-generation wireless communication devices. With the global rollout of 5G and the aggressive research into 6G standards, there is growing demand for materials that can efficiently manipulate electromagnetic waves at terahertz frequencies. Companies such as Rohde & Schwarz are advancing high-frequency testing platforms, indirectly driving innovation in resonance material engineering to meet the stringent requirements of ultra-fast, low-latency communications.
In parallel, the acoustics sector is experiencing a surge in demand for advanced resonant materials that enable precise sound manipulation. This is particularly relevant for applications in noise-canceling architecture, automotive soundproofing, and smart consumer devices. Manufacturers like Bose Corporation are exploring the deployment of engineered resonance materials in their next wave of adaptive acoustic panels and audio systems, aiming for higher fidelity and energy efficiency.
The aerospace and defense sectors are also primed for transformation as Zimmermannian materials promise superior vibration damping and structural adaptability. Firms such as The Boeing Company and Lockheed Martin Corporation are investing in materials research for lighter, more resilient airframes and satellite components that leverage resonance effects for both weight savings and enhanced durability.
Looking ahead to 2030, the outlook for ZRME is marked by opportunities in large-scale smart infrastructure projects. As cities worldwide prioritize sustainability and resilience, the deployment of resonance-engineered composites in bridges, buildings, and transportation networks could improve structural health monitoring and adaptive response to environmental stressors. Early pilot programs are being scoped by industry leaders such as Siemens AG, with a focus on digital integration and real-time performance analytics.
In sum, the next five years will likely see Zimmermannian Resonance Material Engineering transition from specialized research to broader commercial adoption, underpinning disruptive innovations across communications, acoustics, aerospace, and smart infrastructure.
Sources & References
- BASF
- Siemens
- ASTM International
- Henkel
- IBM
- Boeing
- Airbus
- International Organization for Standardization
- European Committee for Standardization
- Murata Manufacturing Co., Ltd.
- KYOCERA Corporation
- CrystalTechno
- Hitachi
- Sumitomo Chemical
- Evonik Industries
- Covestro
- Rohde & Schwarz
- Bose Corporation
- The Boeing Company
- Lockheed Martin Corporation