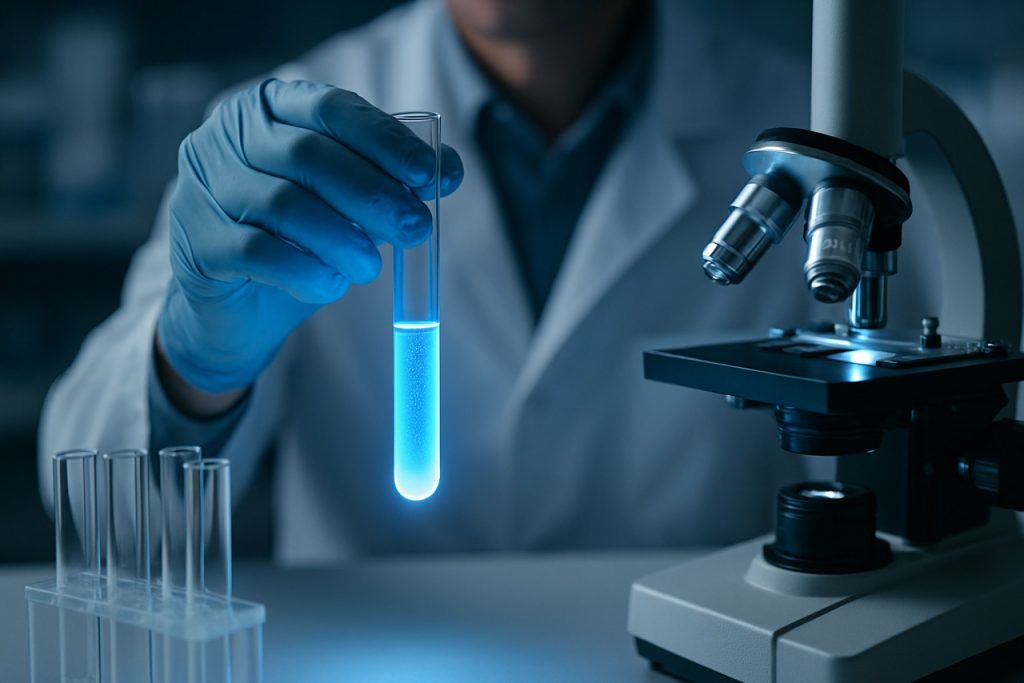
Table of Contents
- Executive Summary: Industry at a Turning Point
- Market Size and Growth Projections Through 2030
- Key Drivers Fueling Adoption in 2025
- Core Technologies and Recent Breakthroughs
- Competitive Landscape: Major Players and Emerging Innovators
- Regulatory Landscape and Industry Standards
- Strategic Partnerships and Investment Trends
- Applications Across Energy, Chemicals, and Materials
- Challenges, Risks, and Barriers to Scale
- Future Outlook: Disruptive Opportunities and Long-Term Impact
- Sources & References
Executive Summary: Industry at a Turning Point
As of 2025, the field of negative electron transfer catalysis—central to emerging energy storage, organic synthesis, and environmental remediation technologies—is at a pivotal juncture. The maturation of electrocatalytic methodologies that exploit negative electron transfer (i.e., reductive catalysis) has accelerated due to advances in catalyst design, process integration, and growing demand for sustainable chemical manufacturing. Key industry players and research consortia are rapidly scaling laboratory successes into pilot and commercial-scale demonstrations, particularly where energy efficiency and selective transformations are critical.
The current year has witnessed a marked increase in corporate and academic partnerships aiming to overcome legacy challenges of catalyst stability and broad substrate compatibility. For instance, several collaborations are underway to integrate negative electron transfer catalysts within electrochemical CO2 reduction systems, with the objective of producing value-added chemicals from waste streams. Major chemical manufacturers and specialized catalyst suppliers are actively expanding their portfolios in this area, leveraging proprietary ligand environments and transition metal complexes that promote high turnover frequencies and selectivity under mild conditions.
Electrification of chemical processes is another driving trend. Companies specializing in electrochemical equipment and green hydrogen infrastructure are investing in negative electron transfer catalysis to enhance the viability of power-to-chemicals pathways. These approaches promise lower carbon footprints and operational costs, aligning with tightening regulatory standards and corporate sustainability commitments. Early-stage commercial deployments, especially in Europe and East Asia, are expected to deliver critical data on process economics and lifecycle performance over the next two years.
Looking forward, the outlook for negative electron transfer catalysis is robust. Industry analysts expect continued growth, driven by the decarbonization of industrial chemistry and the advent of distributed chemical manufacturing. Strategic investments in R&D, intellectual property, and pilot facilities by sector leaders position the industry for breakthroughs in selectivity, scalability, and integration with renewable energy systems. As global demand for climate-neutral products rises, negative electron transfer catalysis stands poised to redefine value chains in chemicals, fuels, and materials, with key stakeholders such as BASF, SABIC, and DuPont signaling increased commitment to this transformative technology.
Market Size and Growth Projections Through 2030
Negative electron transfer catalysis (NETC) is gaining momentum as a transformative approach in chemical manufacturing, energy conversion, and environmental remediation. As of 2025, the market for NETC remains in an early, innovation-driven stage, with substantial investments focused on R&D and pilot-scale deployments. Leading chemical and materials companies are actively exploring NETC for applications such as electrocatalytic reduction of greenhouse gases, selective organic synthesis, and advanced battery chemistries.
Global market size estimates for NETC remain nascent due to the technology’s emerging status, but the sector is expected to experience a compound annual growth rate (CAGR) exceeding 25% through 2030, driven by increased adoption in green chemistry and sustainable manufacturing. This projection is supported by strategic investments from major players in catalysis and electrochemistry, such as BASF, SABIC, and Dow, all of which have announced new initiatives in electrochemical and redox catalysis as part of their sustainability roadmaps.
By 2025, commercial activity is focused mainly on demonstration and pilot projects, with a few early-stage deployments in sectors like specialty chemicals, where NETC enables more efficient and selective transformations than traditional catalytic methods. Companies such as Umicore and 3M are exploring NETC for applications in energy storage and electrocatalytic devices. The rapid expansion of partnerships between academic institutions and industry—exemplified by collaborations supported by organizations such as the National Renewable Energy Laboratory—is also accelerating the pathway to commercialization.
Looking forward to 2030, the NETC market is anticipated to reach a multi-billion-dollar valuation, underpinned by regulatory pressure for carbon-neutral technologies and the increasing economic viability of electrochemical processes. Growth will likely be strongest in Asia-Pacific and Europe, where climate policy and industrial innovation are closely aligned. Key challenges to market expansion include scaling up production, securing stable supply chains for advanced catalyst materials, and further reducing energy consumption in NETC-enabled processes.
In summary, negative electron transfer catalysis is poised for robust growth over the next five years, transitioning from laboratory innovation to commercial reality. The period through 2030 will be critical, as industry leaders and technology developers move from pilot projects to full-scale manufacturing, setting the stage for NETC to become a pillar of sustainable industrial chemistry.
Key Drivers Fueling Adoption in 2025
Negative electron transfer catalysis, a process whereby catalysts facilitate chemical transformations by accepting electrons (i.e., acting as oxidants), is gaining considerable traction in both industrial and academic sectors. Several key drivers are fueling its adoption in 2025 and are likely to shape the market over the next few years.
-
Decarbonization and Green Chemistry Initiatives:
Ongoing global efforts to reduce carbon emissions and transition to sustainable manufacturing are primary drivers. Negative electron transfer catalysis supports milder, less energy-intensive processes for oxidation, enabling industries to reduce their environmental footprint. Companies in specialty chemicals and materials, such as BASF and Dow, have publicly emphasized investments in catalytic technologies that align with sustainability goals. -
Advances in Electrosynthesis and Electrochemical Technologies:
The surge in electrosynthesis—where electricity replaces stoichiometric chemical oxidants—is directly tied to negative electron transfer catalysis. By leveraging renewable electricity, manufacturers are developing scalable and cost-effective oxidation processes. Firms like Siemens are actively integrating electrochemical solutions into industrial workflows, a trend expected to accelerate through 2025 as grid decarbonization deepens. -
Enhanced Selectivity and Efficiency:
Catalysts enabling negative electron transfer have demonstrated higher selectivity in complex transformations, lowering byproduct formation and improving yields. This is particularly valuable in pharmaceuticals, agrochemicals, and fine chemicals. Companies such as Evonik Industries are investing in advanced catalyst platforms to realize these benefits at commercial scale. -
Regulatory Pressures and Supply Chain Security:
Stricter environmental regulations in major markets—including the EU’s Green Deal and evolving U.S. EPA standards—are pushing industries to adopt cleaner catalytic processes. Moreover, negative electron transfer catalysis often allows the use of more abundant, less hazardous feedstocks, helping stabilize supply chains and reduce dependency on critical raw materials—a strategic focus for organizations like Shell and SABIC.
Looking ahead, the convergence of sustainability imperatives, technological innovation, and regulatory momentum will continue to drive adoption. As catalyst manufacturers and end users recognize the dual benefits of operational efficiency and compliance, negative electron transfer catalysis is poised for rapid growth, with pilot projects scaling to commercial deployment between 2025 and 2027.
Core Technologies and Recent Breakthroughs
Negative electron transfer (NET) catalysis is rapidly emerging as a transformative approach within the field of redox catalysis, offering innovative pathways for challenging organic reactions. The core technology leverages catalysts—frequently based on transition metals or organic frameworks—that can facilitate the transfer of electrons in a direction opposite to the conventional flow, enabling the activation of substrates that are typically inert under standard redox conditions. In 2025, significant advancements are being observed, particularly in catalyst design, scalability, and integration with renewable energy systems.
Key breakthroughs have centered around the development of highly selective and robust NET catalysts that operate under mild conditions. For example, the use of tailored ruthenium and nickel complexes has demonstrated superior efficiency in reductive coupling and C–H bond activation processes. Companies such as BASF and Evonik Industries are actively exploring proprietary catalysts capable of facilitating NET processes, aiming to reduce energy consumption and minimize byproduct formation. These industry leaders have reported improved yields and selectivity in pilot-scale applications, particularly for pharmaceutical and fine chemical synthesis, signifying a move towards commercial viability.
Another recent milestone is the integration of NET catalysis with electrochemical and photochemical platforms. By coupling NET catalysts with advanced electrode materials and light-harvesting assemblies, researchers are achieving unprecedented control over reaction kinetics and selectivity. In this context, companies like Merck KGaA have disclosed ongoing projects focused on integrating photoredox modules with NET systems to enable sustainable chemical manufacturing. These hybrid approaches are expected to scale up efficiently, reducing the reliance on hazardous reagents and aligning with green chemistry principles.
Looking ahead to 2025 and beyond, the focus within the sector is shifting towards expanding substrate scope and enhancing catalyst recyclability. Collaboration between industry and academia is intensifying, with consortia and open innovation platforms spearheaded by manufacturers such as DSM and Dow supporting the translation of NET catalysis from laboratory discovery to industrial implementation. Furthermore, advances in computational modeling and in situ spectroscopic techniques are expected to accelerate the rational design of next-generation NET catalysts, promising further breakthroughs in efficiency and sustainability over the next several years.
Competitive Landscape: Major Players and Emerging Innovators
The competitive landscape of negative electron transfer catalysis is rapidly evolving as the field moves from academic research into practical applications across chemical manufacturing, energy, and environmental sectors. As of 2025, established chemical companies are increasingly engaging in this technology, either through internal R&D or partnerships with academic institutions and startups.
Among the leading industry players, BASF SE is noteworthy for its sustained investment in advanced catalysis platforms, including electron transfer processes for green chemistry and novel synthesis pathways. Similarly, SABIC has expanded its portfolio to include research on electron transfer catalysts aimed at improving process efficiency and reducing energy consumption in petrochemical production. Both companies have publicized collaborative research efforts and patent filings in this domain, positioning themselves at the forefront of industrial adoption.
In the energy sector, Shell is actively exploring electron transfer catalysis as part of its broader decarbonization strategy. This includes pilot projects focused on electrocatalytic reduction of CO2 and the synthesis of value-added chemicals from waste streams, leveraging negative electron transfer mechanisms to drive selectivity and efficiency. These projects have gained momentum in 2024–2025, with further scale-ups anticipated in the near term.
Emerging innovators are also shaping the competitive landscape. Startups such as hte GmbH, a subsidiary of BASF, are providing high-throughput catalyst testing and screening services, enabling rapid discovery and optimization of negative electron transfer catalysts. These platforms are facilitating accelerated technology transfer from laboratory to industry, particularly in fine chemicals and pharmaceuticals.
Looking ahead, the competitive landscape is expected to intensify as more multinational chemical firms and agile startups enter the arena, spurred by growing demand for sustainable catalysis. Strategic alliances between technology developers and end-users, as well as increased investment in pilot-scale demonstrations, are likely in the next few years. Intellectual property activity—particularly around novel ligand frameworks and catalyst supports—will be a key differentiator, with companies vying for market leadership through proprietary solutions. The path to commercialization will depend on addressing scalability and cost, but the current trajectory suggests negative electron transfer catalysis is set to become a core technology in the evolving catalysis market.
Regulatory Landscape and Industry Standards
Negative electron transfer catalysis, a rapidly emerging paradigm in chemical synthesis and electrochemical applications, is beginning to attract regulatory attention as its adoption accelerates in 2025. The unique mechanism—where catalysts promote the transfer of electrons in the negative direction—enables new types of redox transformations with broad implications for pharmaceuticals, materials, and environmental remediation. However, the regulatory landscape and industry standards remain in a nascent phase, with oversight primarily adapting from broader catalysis and electrochemical process frameworks.
In the United States, the U.S. Environmental Protection Agency (EPA) continues to update its guidelines on chemical manufacturing, focusing on process safety, environmental emissions, and the toxicity profiles of new catalysts. Although no explicit standards yet govern negative electron transfer catalysis, companies utilizing these methods in fine chemical or pharmaceutical production must comply with existing EPA frameworks, particularly under the Toxic Substances Control Act (TSCA). The EPA has signaled interest in gathering data on new catalytic materials, especially those involving transition metals or novel ligands, for potential future regulation.
In the European Union, the European Chemicals Agency (ECHA) continues to implement the REACH regulation, which requires comprehensive registration, evaluation, and authorization of substances, including new catalysts. As negative electron transfer catalysts often involve previously unregistered compounds, manufacturers and importers must submit detailed dossiers regarding their physicochemical properties and potential hazards. The ECHA has also initiated consultations with industry stakeholders to assess whether specific guidance is needed for advanced catalytic processes, with updates expected over the next several years.
On the industry standards front, organizations such as the International Organization for Standardization (ISO) are beginning exploratory work on harmonized protocols for catalytic performance evaluation and safety assessment. While there are no ISO standards specifically for negative electron transfer catalysis as of 2025, broader standards such as ISO 9001 (quality management) and ISO 14001 (environmental management) are widely adopted by catalyst producers and users. Ongoing discussions at ISO technical committees suggest that draft guidelines specific to advanced catalysis may be proposed as adoption grows.
Looking ahead, regulatory agencies and standards bodies are expected to intensify their focus on negative electron transfer catalysis as commercial applications expand. Priority areas include lifecycle analysis, end-of-life catalyst management, and harmonization of safety and reporting protocols across jurisdictions. As industry groups and regulatory authorities collaborate, the next few years will likely see the emergence of more tailored regulatory frameworks and industry standards designed to ensure safe, sustainable, and responsible deployment of this innovative technology.
Strategic Partnerships and Investment Trends
Strategic partnerships and investments within the field of negative electron transfer catalysis (NETC) have witnessed significant momentum entering 2025, as stakeholders recognize the technology’s potential to enable more energy-efficient chemical transformations, advanced battery systems, and sustainable synthesis routes. Collaboration between academia, established chemical manufacturers, and emerging deep-tech startups is accelerating the commercialization of NETC platforms, especially as the sector shifts focus from proof-of-concept studies to scalable applications.
A notable trend is the formation of alliances between large multinational chemical companies and research-driven startups specializing in electron transfer catalysis. For instance, companies like BASF and Dow have intensified their investment in advanced catalysis research, establishing joint ventures and sponsoring academic-industry research consortia to de-risk the translation of NETC from laboratory to industrial scale. These partnerships are often structured to combine the startups’ innovative molecular design capabilities with the established process engineering and global infrastructure of the larger firms.
Venture capital investment in NETC-focused startups has also grown, with deep-tech funds and corporate venture arms targeting companies developing novel catalysts, electrochemical cells, and process integration solutions. Strategic investments are increasingly aimed at accelerating pilot demonstrations and early commercial deployment, particularly for applications in CO2 reduction, nitrogen fixation, and organic synthesis. Notably, Arkema and Linde have both announced targeted funding initiatives for next-generation catalysis, including support for NETC-related projects that promise significant reductions in carbon intensity and energy consumption.
Government and intergovernmental organizations are also playing a catalytic role. Public-private partnerships, supported by agencies such as the U.S. Department of Energy, are facilitating pre-competitive research and the creation of open innovation platforms. These efforts aim to lower entry barriers for small enterprises and academic groups, thereby broadening the innovation ecosystem and fostering cross-sector collaboration.
Looking ahead, experts anticipate that strategic partnerships will further extend to cover end-users in pharmaceuticals, energy storage, and sustainable materials. As NETC technologies mature and regulatory incentives for decarbonization intensify, the sector is poised for increased cross-border investment and the emergence of global innovation hubs dedicated to electron transfer catalysis. This collaborative framework is expected to accelerate commercialization pathways and establish NETC as a foundational platform for sustainable chemistry in the coming years.
Applications Across Energy, Chemicals, and Materials
Negative electron transfer (NET) catalysis, wherein catalysts facilitate electron transfer in the negative direction (i.e., drive energetically uphill electron flow), is emerging as a transformative approach for a range of applications spanning the energy, chemical, and materials sectors. In 2025, the deployment of NET catalysis is accelerating, driven by advances in catalyst design, operando characterization, and scalable synthesis methods.
One of the most prominent application areas is in the field of sustainable energy. NET catalysis is being integrated into next-generation electrochemical devices for energy storage and conversion. For example, in CO2 electroreduction, NET catalysts enable the selective formation of high-value chemicals such as ethylene and ethanol from CO2, directly addressing the challenge of carbon utilization. Industrial leaders like BASF and SABIC are actively exploring NET-catalyzed processes for closed-loop carbon cycles, aiming to reduce net greenhouse gas emissions in chemical manufacturing.
In the chemicals sector, NET catalysis allows for unconventional bond activations, including C–H and C–O bond functionalization under mild conditions. This is fostering new synthetic methodologies for fine and specialty chemicals, including pharmaceuticals and agrochemicals. Companies such as Dow are investing in the development of electrocatalytic reactors that exploit NET principles to enhance reaction selectivity and energy efficiency.
Materials science is also seeing significant advancements due to NET catalysis, particularly in the synthesis of advanced polymers and nanomaterials. NET-enabled polymerizations offer precise control over molecular weight and architecture, which is critical for high-performance materials used in automotive, aerospace, and electronics. Arkema and DuPont are among the companies piloting NET-catalyzed processes for next-generation polymer production, with an emphasis on recyclability and reduced environmental impact.
Looking ahead, the outlook for NET catalysis in these sectors is highly promising. Major industry players are scaling up pilot projects, while partnerships between academia and industry are accelerating technology transfer. As NET catalysis matures, its integration with digital process controls and renewable energy sources is expected to further enhance sustainability and cost-effectiveness, positioning NET as a cornerstone technology for the chemicals and materials industries in the coming years.
Challenges, Risks, and Barriers to Scale
Negative electron transfer (NET) catalysis, an emerging area in chemical synthesis and energy conversion, faces a series of challenges, risks, and barriers as it moves towards large-scale application in 2025 and the following years. Despite remarkable academic advances, several hurdles must be overcome for NET catalysis to realize its industrial potential.
A principal challenge lies in catalyst development and stability. Many NET catalysts, often based on transition metal complexes or organic molecules, exhibit limited operational lifetimes under industrial conditions, such as elevated temperatures, strong solvents, or prolonged cycling. The propensity for catalyst deactivation or structural degradation poses a substantial barrier to consistent, long-term use. Companies focusing on advanced materials, such as BASF and Arkema, are investing in research to improve catalyst robustness, but the gap between laboratory performance and real-world demands remains significant.
Another risk involves the scalability of NET catalysis processes. Many mechanistic insights and breakthroughs have been demonstrated at the milligram or gram scale. However, scaling these processes to kilogram or ton scales introduces issues related to mass transfer, reaction kinetics, and reactor design. For example, the need for precise control over electron transfer events and minimizing side reactions can be difficult to replicate in larger, less controlled environments. Process engineering firms like Siemens and Honeywell are actively developing modular flow reactors and digital process control strategies, but widespread implementation is still in its infancy.
Supply chain and feedstock availability add another layer of complexity. Some NET catalytic systems require rare or geopolitically sensitive metals (e.g., ruthenium, iridium), which could create vulnerabilities in supply and price volatility. This issue is recognized by organizations such as Umicore, which is working to secure sustainable sourcing and recycling pathways, but until widespread alternatives are found, this remains a critical bottleneck.
Finally, regulatory and safety concerns are non-trivial. NET catalysis may involve reactive intermediates, high-energy electron donors, or novel chemicals with uncharacterized toxicological profiles. Regulatory agencies and industrial safety bodies are only beginning to develop guidelines for these systems, potentially delaying permits and increasing compliance costs for early adopters.
Looking to 2025 and beyond, overcoming these challenges will require coordinated efforts between catalyst developers, process engineers, materials suppliers, and regulators. Consortia involving established chemical players (e.g., BASF, Evonik), equipment manufacturers, and public research institutions are anticipated to play a vital role in advancing NET catalysis from the laboratory to industrial scale.
Future Outlook: Disruptive Opportunities and Long-Term Impact
Negative electron transfer catalysis (NETC) is positioned to become a disruptive force in chemical manufacturing and advanced materials synthesis as we advance into 2025 and beyond. The core principle—leveraging catalysts to facilitate electron uptake from substrates, rather than the more common electron donation—unlocks new reactivity paradigms, particularly for activating inert molecules or achieving selective transformations under mild conditions. This paradigm shift is drawing increasing attention from both academic and industrial innovators due to its potential to enable greener, more efficient processes.
In the near term, several industrial stakeholders are accelerating the translation of NETC from proof-of-concept research to scalable applications. For example, companies in the specialty chemicals space, such as BASF and Dow, have announced R&D initiatives aimed at integrating advanced catalytic platforms, including those exploiting negative electron transfer, into next-generation synthesis and polymerization protocols. Efforts are focused on processes such as C–H activation, oxidative coupling, and functionalization of traditionally inert hydrocarbons, where NETC offers efficiency and selectivity advantages.
- Electrochemical Manufacturing: NETC mechanisms are increasingly being embedded in electrochemical reactors to drive sustainable transformations. Companies like DuPont are exploring electro-organic synthesis leveraging NETC to reduce energy consumption and byproduct formation in pharmaceutical and agrochemical synthesis.
- Materials Innovation: In advanced materials, NETC is being investigated for precision doping of semiconductors and in the synthesis of specialty polymers with unique electronic properties. Firms such as SABIC are supporting collaborative projects targeting NETC-derived materials for electronics and energy storage.
- Environmental Impact: The potential of NETC processes to facilitate low-temperature, solvent-minimized synthesis is seen as a route toward reducing the environmental footprint of chemical manufacturing. Organizations including Shell are evaluating NETC as part of their broader decarbonization strategies.
Looking ahead, the next few years are expected to witness increased patent activity and pilot-scale deployments as NETC platforms mature. The intersection with digital chemistry—using machine learning to optimize NETC catalyst design—may further accelerate adoption. If scalability and cost barriers are addressed, NETC could reshape how bulk chemicals, fine chemicals, and advanced materials are produced, with profound economic and sustainability benefits across sectors.