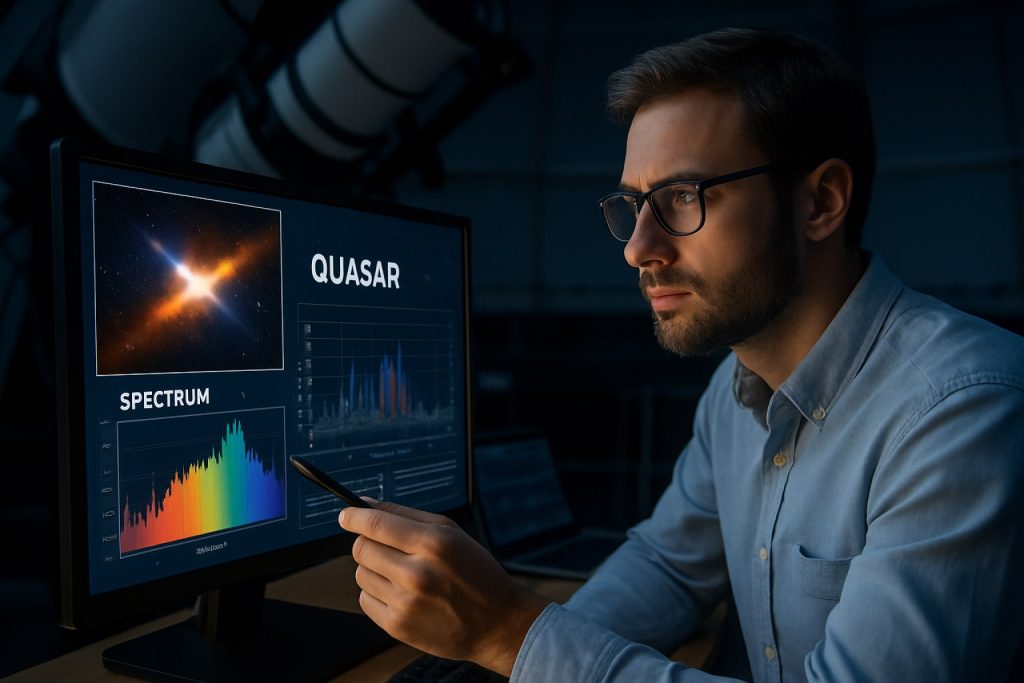
Quasars Research Spectroscopy in 2025: Unveiling the Universe’s Deepest Mysteries with Next-Gen Technologies. Explore How Spectroscopic Innovations Will Transform Quasar Studies and Astrophysical Discoveries Over the Next Five Years.
- Executive Summary: Key Trends and Market Outlook (2025–2029)
- Global Market Forecast: Growth Drivers and Revenue Projections
- Technological Innovations in Quasar Spectroscopy: Instruments and Methods
- Major Industry Players and Strategic Collaborations
- Emerging Applications: From Cosmology to Black Hole Physics
- Data Analysis and AI Integration in Spectroscopic Research
- Regulatory Landscape and Standardization Efforts
- Investment, Funding, and Academic-Industry Partnerships
- Regional Analysis: North America, Europe, Asia-Pacific, and Beyond
- Future Outlook: Challenges, Opportunities, and Roadmap to 2030
- Sources & References
Executive Summary: Key Trends and Market Outlook (2025–2029)
Quasars research spectroscopy is poised for significant advancements between 2025 and 2029, driven by technological innovation, expanded international collaboration, and the commissioning of next-generation observatories. Quasars—extremely luminous active galactic nuclei powered by supermassive black holes—are critical probes of the early universe, and spectroscopy remains the primary tool for unraveling their composition, redshift, and physical processes.
A key trend is the deployment of advanced spectrographs on both ground-based and space-based telescopes. The European Southern Observatory (ESO) is leading efforts with the Extremely Large Telescope (ELT), expected to begin operations in the latter half of the decade. The ELT’s high-resolution spectrographs, such as HIRES, will enable unprecedented studies of quasar absorption lines, intergalactic medium, and chemical evolution at high redshifts. Similarly, the National Aeronautics and Space Administration (NASA) continues to support quasar research through the James Webb Space Telescope (JWST), which, since its 2022 launch, has already delivered transformative infrared spectra of distant quasars and will remain a cornerstone for space-based spectroscopy.
On the instrumentation front, companies like Thorlabs and Carl Zeiss AG are supplying precision optics and spectroscopic components, supporting both custom research instruments and large-scale observatory projects. These manufacturers are investing in improved detector sensitivity, adaptive optics, and calibration systems, which are essential for extracting faint quasar signals from background noise.
Data volume and complexity are also increasing, prompting the adoption of artificial intelligence and machine learning for spectral analysis. Organizations such as European Space Agency (ESA) and National Radio Astronomy Observatory (NRAO) are developing open-access data platforms and automated pipelines to handle the influx of high-resolution spectra, facilitating cross-survey comparisons and accelerating discovery.
Looking ahead, the market outlook for quasars research spectroscopy is robust. Funding from governmental agencies and international consortia is expected to remain strong, with a focus on understanding cosmic reionization, black hole growth, and the evolution of large-scale structure. The integration of multi-wavelength data—from radio to X-ray—will further enhance the scientific return, with collaborations between observatories such as ESO, NASA, and ESA playing a pivotal role. As new facilities come online and analytical techniques mature, the period from 2025 to 2029 is set to yield major breakthroughs in our understanding of quasars and the early universe.
Global Market Forecast: Growth Drivers and Revenue Projections
The global market for quasars research spectroscopy is poised for significant growth in 2025 and the following years, driven by advances in astronomical instrumentation, increased funding for space science, and the expanding capabilities of both ground-based and space-based observatories. Quasars—extremely luminous active galactic nuclei powered by supermassive black holes—are critical to understanding the early universe, cosmic evolution, and the intergalactic medium. Spectroscopy remains the primary tool for probing their properties, redshifts, and chemical compositions.
Key growth drivers include the deployment of next-generation telescopes and spectrographs. The European Southern Observatory (ESO) is advancing the Extremely Large Telescope (ELT), set to become operational in the coming years, which will feature state-of-the-art spectroscopic instruments designed for high-resolution studies of distant quasars. Similarly, the National Aeronautics and Space Administration (NASA) continues to support missions such as the James Webb Space Telescope (JWST), which, with its near- and mid-infrared spectroscopic capabilities, is already yielding unprecedented data on high-redshift quasars and is expected to drive further discoveries through 2025 and beyond.
On the instrumentation side, companies like Thorlabs and Carl Zeiss AG are recognized suppliers of advanced optical components and spectroscopic systems, supporting both research institutions and observatories worldwide. Their ongoing innovation in detectors, gratings, and fiber optics is crucial for enhancing the sensitivity and resolution of spectroscopic measurements, directly impacting the quality and quantity of quasar data collected.
Revenue projections for the quasars research spectroscopy market are closely tied to public and private investments in astronomical infrastructure. The global scientific instrumentation sector, which includes spectroscopy, is expected to see steady growth, with a compound annual growth rate (CAGR) in the mid-single digits through the late 2020s, as reported by industry participants. The increasing number of international collaborations, such as those coordinated by ESO and NASA, is also expanding the market for specialized spectroscopic equipment and software.
Looking ahead, the outlook for 2025 and the next few years is robust. The commissioning of new observatories, the launch of additional space telescopes, and the continuous improvement of spectroscopic technologies are expected to drive both scientific breakthroughs and market expansion. As the demand for high-precision quasar data grows, suppliers and research organizations are well-positioned to benefit from increased funding and collaborative opportunities in this dynamic field.
Technological Innovations in Quasar Spectroscopy: Instruments and Methods
The field of quasar research spectroscopy is experiencing a period of rapid technological advancement, driven by the deployment of next-generation telescopes and the development of highly sensitive spectrographs. As of 2025, several major observatories and instrument manufacturers are at the forefront of these innovations, enabling astronomers to probe the distant universe with unprecedented precision.
A key milestone is the operational status of the European Southern Observatory‘s Extremely Large Telescope (ELT), which is expected to deliver first light in the coming years. The ELT will be equipped with advanced spectrographs such as HIRES and MOSAIC, designed to capture high-resolution spectra of faint and distant quasars. These instruments will allow researchers to study the chemical composition, kinematics, and intergalactic environments of quasars at redshifts previously inaccessible, providing new insights into the early universe.
Similarly, the Gemini Observatory is enhancing its spectroscopic capabilities with the Gemini High-resolution Optical SpecTrograph (GHOST), which began science operations in late 2023. GHOST offers high throughput and broad wavelength coverage, making it ideal for detailed studies of quasar absorption lines and the intergalactic medium. The instrument’s design emphasizes stability and precision, crucial for detecting subtle features in quasar spectra.
On the space-based front, the National Aeronautics and Space Administration (NASA)’s James Webb Space Telescope (JWST) continues to revolutionize infrared spectroscopy of quasars. Its Near Infrared Spectrograph (NIRSpec) and Mid-Infrared Instrument (MIRI) are enabling the detection of dust-obscured quasars and the characterization of their host galaxies at high redshift. The JWST’s sensitivity is opening new windows into the epoch of reionization and the growth of supermassive black holes.
Looking ahead, the National Astronomical Observatory of Japan (NAOJ) is preparing for the Thirty Meter Telescope (TMT), which will feature state-of-the-art spectrographs for both optical and near-infrared observations. The TMT’s large aperture and adaptive optics systems are expected to further enhance the study of faint quasars and their environments.
In addition to hardware, advances in data processing and machine learning are playing a growing role. Automated pipelines and AI-driven analysis tools are being developed to handle the massive data volumes generated by these instruments, enabling faster and more accurate extraction of quasar spectral features.
Overall, the next few years promise significant breakthroughs in quasar spectroscopy, driven by the synergy of cutting-edge instrumentation, international collaboration, and computational innovation. These developments are poised to deepen our understanding of the universe’s most luminous and distant objects.
Major Industry Players and Strategic Collaborations
The field of quasars research spectroscopy is experiencing significant momentum in 2025, driven by the collaborative efforts of major industry players and leading astronomical organizations. These entities are leveraging advanced spectroscopic technologies to unravel the mysteries of quasars—extremely luminous active galactic nuclei powered by supermassive black holes. The synergy between public research institutions, private companies, and international consortia is shaping the current landscape and setting the stage for breakthroughs in the coming years.
A central role is played by European Southern Observatory (ESO), which operates some of the world’s most advanced ground-based telescopes, including the Very Large Telescope (VLT) and the upcoming Extremely Large Telescope (ELT). ESO’s spectrographs, such as X-shooter and ESPRESSO, are at the forefront of high-resolution quasar spectroscopy, enabling detailed studies of the intergalactic medium and the early universe. The ELT, expected to see first light in the next few years, is anticipated to revolutionize quasar research with its unprecedented light-gathering power and next-generation spectrographs.
In the United States, NOIRLab (National Optical-Infrared Astronomy Research Laboratory) coordinates access to key facilities like the Gemini Observatory and the upcoming Vera C. Rubin Observatory. These observatories are integral to large-scale spectroscopic surveys, such as the Dark Energy Spectroscopic Instrument (DESI) project, which is mapping millions of quasars to probe cosmic expansion and structure formation.
On the industry side, companies specializing in high-precision optics and spectrograph manufacturing are crucial collaborators. Carl Zeiss AG and Thorlabs, Inc. are recognized for supplying advanced optical components and custom spectroscopic solutions to both research institutions and observatories worldwide. Their innovations in adaptive optics, diffraction gratings, and detector technologies are directly enhancing the sensitivity and accuracy of quasar spectroscopy instruments.
Strategic collaborations are also evident in international consortia, such as the Square Kilometre Array (SKA) project, which includes partners from Europe, Africa, Asia, and Australia. While primarily a radio observatory, SKA’s synergy with optical spectroscopic data is expected to provide a multi-wavelength perspective on quasar environments and evolution.
Looking ahead, the next few years will see deeper integration between hardware manufacturers, data analytics firms, and astronomical research bodies. This collaborative ecosystem is poised to accelerate discoveries in quasar physics, cosmology, and the fundamental laws governing the universe.
Emerging Applications: From Cosmology to Black Hole Physics
Quasars, among the brightest and most distant objects in the universe, continue to be pivotal in advancing both cosmology and black hole physics. Spectroscopy remains the primary tool for probing their properties, enabling researchers to dissect the light from these active galactic nuclei and extract information about their composition, redshift, and the environments surrounding supermassive black holes. In 2025 and the coming years, several major initiatives and technological advancements are set to expand the frontiers of quasar research spectroscopy.
A significant development is the deployment and operation of next-generation telescopes equipped with advanced spectrographs. The European Southern Observatory (ESO) is leading efforts with the Extremely Large Telescope (ELT), which will feature high-resolution spectroscopic instruments designed to analyze faint and distant quasars. The ELT’s spectrographs, such as HIRES and MOSAIC, are expected to deliver unprecedented sensitivity and resolution, allowing for detailed studies of quasar emission and absorption lines. These capabilities will help refine measurements of cosmic expansion, probe the intergalactic medium, and test fundamental physics, such as the constancy of physical constants over cosmic time.
In parallel, the James Webb Space Telescope (JWST), operated by NASA, is now providing infrared spectroscopic data on high-redshift quasars, revealing the conditions in the early universe and the growth of the first supermassive black holes. JWST’s NIRSpec and MIRI instruments are enabling the detection of molecular and atomic features previously inaccessible, offering new insights into quasar host galaxies and their environments.
Ground-based facilities are also advancing. The National Optical-Infrared Astronomy Research Laboratory (NOIRLab) in the United States is supporting large-scale spectroscopic surveys, such as the Dark Energy Spectroscopic Instrument (DESI), which is mapping millions of quasars to trace the large-scale structure of the universe and constrain dark energy models. These surveys are expected to yield vast datasets, fostering machine learning applications for automated quasar classification and redshift determination.
Looking ahead, the synergy between these observatories and upcoming projects like the Vera C. Rubin Observatory (operated by the LSST Corporation) will further enhance time-domain spectroscopy, enabling the study of quasar variability and transient phenomena linked to black hole accretion events. As data volumes grow, collaborations with technology providers specializing in high-throughput detectors and data processing—such as Carl Zeiss AG and Hamamatsu Photonics—will be crucial for maximizing scientific returns.
In summary, the period from 2025 onward promises transformative progress in quasar research spectroscopy, with emerging applications ranging from precision cosmology to unraveling the physics of supermassive black holes, driven by international collaboration and technological innovation.
Data Analysis and AI Integration in Spectroscopic Research
The integration of advanced data analysis techniques and artificial intelligence (AI) is rapidly transforming the field of quasar research spectroscopy as of 2025. The vast datasets generated by modern spectroscopic surveys—often comprising millions of spectra—necessitate robust, automated methods for data processing, feature extraction, and anomaly detection. AI-driven approaches, particularly those leveraging machine learning and deep learning, are now central to handling this data deluge and extracting meaningful astrophysical insights.
Major astronomical observatories and research collaborations are at the forefront of this transformation. The European Southern Observatory (ESO) continues to operate and upgrade instruments such as the Very Large Telescope (VLT), which produces high-resolution quasar spectra. These datasets are increasingly analyzed using AI algorithms to classify quasar types, identify redshifted emission lines, and detect subtle spectral features indicative of intervening matter or black hole activity. Similarly, the National Optical-Infrared Astronomy Research Laboratory (NOIRLab) in the United States supports large-scale surveys like the Dark Energy Spectroscopic Instrument (DESI), which relies on automated pipelines and machine learning for real-time data reduction and target identification.
In 2025, the deployment of next-generation telescopes and spectrographs is further accelerating the adoption of AI. The ESO’s Extremely Large Telescope (ELT), expected to begin science operations soon, will generate unprecedented volumes of high-fidelity quasar spectra. To manage and interpret this data, research teams are developing custom AI models capable of unsupervised clustering, anomaly detection, and automated parameter estimation. These models are trained on both simulated and archival data, ensuring robust performance across diverse quasar populations.
Cloud-based platforms and open-source software frameworks are also playing a pivotal role. Initiatives such as the Vera C. Rubin Observatory’s Legacy Survey of Space and Time (LSST) are making their data products and analysis tools accessible to the global community, fostering collaborative development of AI-driven spectroscopic analysis pipelines. These efforts are supported by partnerships with technology providers and academic institutions, ensuring that the latest advances in AI are rapidly translated into practical tools for quasar research.
Looking ahead, the outlook for data analysis and AI integration in quasar spectroscopy is highly promising. As instruments become more sensitive and datasets grow in complexity, the synergy between astronomy and AI is expected to yield new discoveries about quasar evolution, the intergalactic medium, and the nature of supermassive black holes. The continued investment by organizations such as ESO, NOIRLab, and Vera C. Rubin Observatory will be instrumental in shaping the future of this dynamic research area.
Regulatory Landscape and Standardization Efforts
The regulatory landscape and standardization efforts in quasars research spectroscopy are evolving rapidly as the field matures and as new, more sensitive instruments come online. In 2025, the focus is on harmonizing data collection, calibration, and sharing protocols to ensure reproducibility and interoperability across international research collaborations. This is particularly important as large-scale observatories and space missions generate vast amounts of spectroscopic data on quasars, requiring robust frameworks for data management and quality assurance.
Key organizations such as the European Southern Observatory (ESO) and the National Aeronautics and Space Administration (NASA) are at the forefront of these efforts. ESO, for example, operates the Very Large Telescope (VLT) and is developing the Extremely Large Telescope (ELT), both of which are equipped with advanced spectrographs designed for high-precision quasar observations. These facilities adhere to strict calibration standards and data formats, which are increasingly being adopted as benchmarks by the global community.
In parallel, the International Astronomical Union (IAU) continues to play a central role in coordinating standardization initiatives. The IAU’s Commission B5 on Documentation and Astronomical Data is actively working on guidelines for spectroscopic data archiving, metadata standards, and best practices for open data sharing. These guidelines are expected to be updated in 2025 to reflect the latest technological advancements and the growing importance of machine learning in data analysis.
Another significant development is the increasing involvement of space agencies such as the Japan Aerospace Exploration Agency (JAXA) and the European Space Agency (ESA), both of which are launching or supporting missions with dedicated spectroscopic capabilities for quasar research. These agencies are collaborating on cross-calibration exercises and joint data repositories, further driving the need for harmonized standards.
Looking ahead, the next few years are likely to see the formalization of international standards for quasar spectroscopy, particularly as the ELT and NASA’s Nancy Grace Roman Space Telescope come online. These instruments will set new benchmarks for data quality and volume, necessitating even more rigorous regulatory frameworks. The outlook is for increased transparency, interoperability, and reproducibility, with regulatory bodies and standardization organizations working closely with observatories and instrument manufacturers to ensure that the rapidly expanding field of quasar spectroscopy remains robust and scientifically credible.
Investment, Funding, and Academic-Industry Partnerships
Investment and funding in the field of quasars research spectroscopy have seen a notable uptick as both public and private stakeholders recognize the scientific and technological significance of understanding these distant cosmic phenomena. In 2025, major government agencies such as the National Aeronautics and Space Administration (NASA) and the European Space Agency (ESA) continue to allocate substantial resources to space-based observatories and ground-based telescope upgrades, with a focus on high-resolution spectroscopic instruments. NASA’s ongoing support for missions like the James Webb Space Telescope (JWST) and the upcoming Nancy Grace Roman Space Telescope underscores the agency’s commitment to advancing spectroscopic capabilities for quasar studies.
On the industry side, leading optical and photonics companies such as Carl Zeiss AG and Thorlabs, Inc. are actively collaborating with academic institutions to develop next-generation spectrographs and detectors. These partnerships are often facilitated through joint grant applications, technology transfer agreements, and co-development projects. For example, Zeiss has a long-standing history of supplying precision optics for astronomical instrumentation, while Thorlabs is known for its modular spectroscopy solutions that are increasingly being adopted in research observatories.
Academic-industry partnerships are further bolstered by international consortia such as the European Southern Observatory (ESO), which coordinates multi-institutional efforts to design and deploy advanced spectroscopic instruments on telescopes like the Very Large Telescope (VLT) and the upcoming Extremely Large Telescope (ELT). These collaborations often involve shared funding models, where industry partners contribute technology and expertise, while academic groups provide scientific leadership and data analysis capabilities.
In terms of funding trends, philanthropic organizations and private foundations are increasingly entering the field. The Alfred P. Sloan Foundation and the Simons Foundation have both announced new grant programs in 2024-2025 aimed at supporting large-scale spectroscopic surveys of quasars, with an emphasis on open data and cross-disciplinary research.
Looking ahead, the outlook for investment and partnerships in quasars research spectroscopy remains robust. The anticipated commissioning of new facilities, such as the ELT and the Vera C. Rubin Observatory, is expected to drive further collaboration between academia and industry, particularly in the development of custom spectroscopic instrumentation and data processing pipelines. As the demand for high-precision, high-throughput spectroscopy grows, the sector is likely to see continued inflows of capital and expertise from both traditional and emerging stakeholders.
Regional Analysis: North America, Europe, Asia-Pacific, and Beyond
Quasars research spectroscopy continues to be a dynamic field across North America, Europe, Asia-Pacific, and other regions, with each contributing unique capabilities and infrastructure to advance the understanding of these distant cosmic phenomena. As of 2025, the regional landscape is shaped by major observatories, collaborative projects, and the deployment of next-generation spectroscopic instruments.
North America remains at the forefront, driven by the resources and expertise of institutions such as the National Aeronautics and Space Administration (NASA) and the National Optical-Infrared Astronomy Research Laboratory (NOIRLab). The Gemini Observatory, with its twin telescopes in Hawaii and Chile, continues to provide high-resolution spectroscopic data on quasars, enabling studies of their emission lines and intervening intergalactic medium. The Vera C. Rubin Observatory, expected to begin full science operations in 2025, will further enhance time-domain spectroscopy, allowing researchers to monitor quasar variability and transient phenomena.
In Europe, the European Southern Observatory (ESO) leads with its Very Large Telescope (VLT) and the upcoming Extremely Large Telescope (ELT), both located in Chile. The VLT’s suite of spectrographs, such as X-shooter and ESPRESSO, are central to high-precision measurements of quasar spectra, probing the early universe and the nature of dark matter. The ELT, anticipated to see first light in the next few years, is expected to revolutionize quasar spectroscopy with unprecedented sensitivity and resolution. European collaborations, including the European Space Agency (ESA), also support space-based spectroscopic missions that complement ground-based efforts.
The Asia-Pacific region is rapidly expanding its role. The National Astronomical Observatory of Japan (NAOJ) operates the Subaru Telescope in Hawaii, which is equipped with advanced spectrographs like the Prime Focus Spectrograph (PFS) for large-scale quasar surveys. China’s Chinese Academy of Sciences (CAS) is investing in new facilities and international collaborations, including the Large Sky Area Multi-Object Fiber Spectroscopic Telescope (LAMOST), which has already cataloged thousands of quasars and will continue to do so with upgraded instrumentation.
Beyond these regions, countries such as Australia and India are increasing their participation through projects like the Australian Astronomical Optics (AAO) and the Indian Institute of Astrophysics (IIA). These efforts are often integrated into global networks, ensuring that quasar spectroscopy benefits from a truly international approach. Looking ahead, the synergy between regional observatories and the deployment of new spectroscopic technologies is expected to yield transformative insights into quasar physics and cosmology through 2025 and beyond.
Future Outlook: Challenges, Opportunities, and Roadmap to 2030
The future of quasar research spectroscopy is poised for significant advancements as new observational facilities, instrumentation, and data analysis techniques come online through 2025 and into the next several years. The field faces both technical and scientific challenges, but also unprecedented opportunities to deepen our understanding of the early universe, supermassive black holes, and cosmic evolution.
A major driver of progress is the deployment of next-generation telescopes and spectrographs. The European Southern Observatory (ESO) is advancing the Extremely Large Telescope (ELT), expected to achieve first light in the coming years. With its 39-meter aperture and advanced spectroscopic instruments, the ELT will enable high-resolution studies of faint, distant quasars, probing the epoch of reionization and the growth of the earliest supermassive black holes. Similarly, the Gemini Observatory and NOIRLab are upgrading their facilities with new spectrographs, enhancing sensitivity and wavelength coverage for quasar surveys.
Space-based observatories are also set to play a crucial role. The National Aeronautics and Space Administration (NASA) James Webb Space Telescope (JWST), operational since 2022, is already delivering transformative infrared spectra of high-redshift quasars. Over the next few years, JWST’s capabilities will be complemented by the upcoming European Space Agency (ESA) Euclid mission and NASA’s Nancy Grace Roman Space Telescope, both of which will provide wide-field spectroscopic surveys, enabling statistical studies of quasar populations and their environments.
Despite these advances, several challenges remain. The sheer volume of data from new instruments will require robust data processing pipelines and machine learning algorithms for spectral analysis and anomaly detection. Cross-matching multi-wavelength data from ground and space observatories will be essential for comprehensive quasar characterization. Additionally, atmospheric and instrumental calibration, especially for ground-based near-infrared spectroscopy, will demand ongoing innovation.
Opportunities abound in the synergy between observational and theoretical work. Improved spectroscopic data will refine models of quasar accretion physics, feedback mechanisms, and their role in galaxy evolution. International collaborations, such as those coordinated by International Astronomical Union (IAU), are expected to foster data sharing and joint analysis, accelerating discoveries.
By 2030, the roadmap for quasar research spectroscopy envisions a landscape where high-precision, multi-wavelength spectra of thousands of quasars are routinely obtained and analyzed. This will not only illuminate the nature of quasars themselves but also provide critical insights into the history and structure of the universe.
Sources & References
- European Southern Observatory
- National Aeronautics and Space Administration
- Thorlabs
- Carl Zeiss AG
- European Space Agency
- National Radio Astronomy Observatory
- Gemini Observatory
- National Astronomical Observatory of Japan
- NOIRLab
- LSST Corporation
- Hamamatsu Photonics
- Japan Aerospace Exploration Agency
- Simons Foundation
- National Astronomical Observatory of Japan (NAOJ)
- Chinese Academy of Sciences (CAS)
- Indian Institute of Astrophysics (IIA)