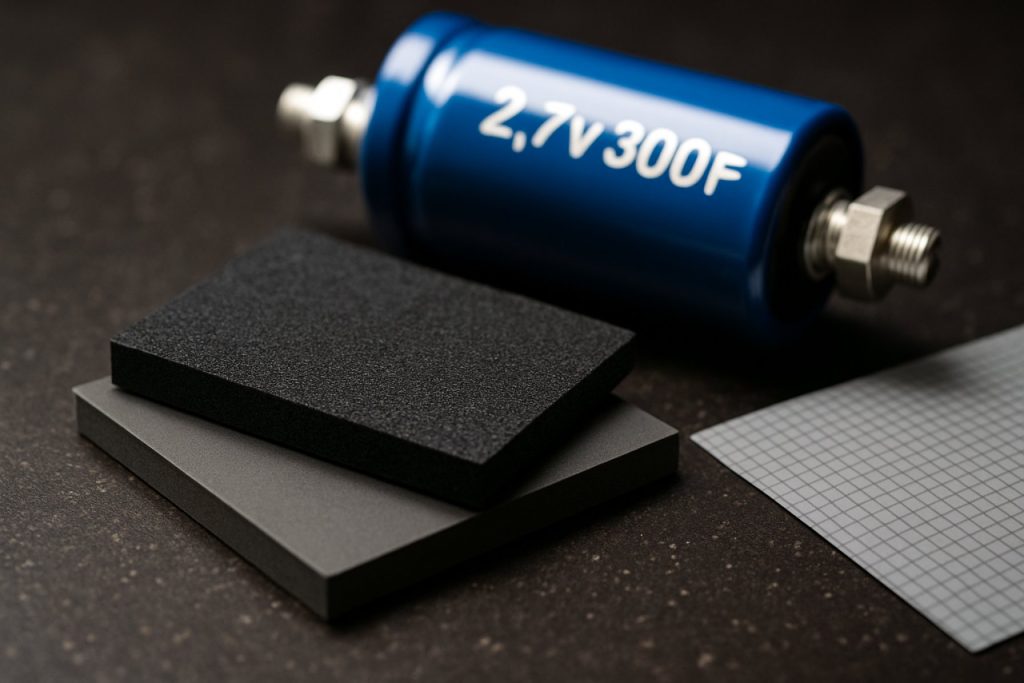
Supercapacitor Electrode Materials Engineering in 2025: Pioneering Next-Gen Energy Storage. Explore the Innovations, Market Dynamics, and Future Growth Shaping the Industry.
- Executive Summary: Key Trends and Market Drivers
- Global Market Size and Forecast (2025–2030): CAGR and Revenue Projections
- Material Innovations: Graphene, Carbon Nanotubes, and Beyond
- Manufacturing Advances and Scalability Challenges
- Performance Metrics: Energy Density, Power Density, and Lifespan
- Key Players and Strategic Partnerships (e.g., Skeleton Technologies, Maxwell Technologies, Panasonic)
- Application Landscape: Automotive, Grid Storage, Consumer Electronics
- Sustainability and Environmental Impact of Electrode Materials
- Regulatory Standards and Industry Initiatives (e.g., ieee.org, sae.org)
- Future Outlook: Disruptive Technologies and Market Opportunities
- Sources & References
Executive Summary: Key Trends and Market Drivers
The supercapacitor sector is undergoing rapid transformation, driven by advances in electrode materials engineering that are unlocking new performance thresholds and broadening application horizons. As of 2025, the industry is witnessing a pronounced shift from traditional activated carbon electrodes toward next-generation materials such as graphene, carbon nanotubes, and hybrid composites. These innovations are enabling higher energy and power densities, longer cycle life, and improved safety profiles, directly addressing the limitations that have historically constrained supercapacitor adoption in sectors like automotive, grid storage, and consumer electronics.
Key industry players are investing heavily in R&D to commercialize advanced electrode materials. Maxwell Technologies, a subsidiary of Tesla, continues to pioneer the integration of proprietary dry electrode technology, aiming to enhance both the scalability and performance of supercapacitor cells. Meanwhile, Skeleton Technologies is leveraging its patented “Curved Graphene” material to deliver ultracapacitors with up to four times the power density of conventional devices, targeting applications in transportation and industrial power management. Panasonic Corporation and Eaton are also expanding their portfolios, focusing on hybrid supercapacitor solutions that blend the high energy density of batteries with the rapid charge-discharge capabilities of capacitors.
The market is further propelled by regulatory and sustainability drivers. The push for electrification in mobility and renewable energy integration is accelerating demand for energy storage systems with fast response times and long operational lifespans. Supercapacitors, with their ability to deliver millions of charge-discharge cycles, are increasingly seen as complementary to lithium-ion batteries, particularly in applications requiring high power bursts or rapid energy recovery. The European Union’s Green Deal and similar initiatives in Asia and North America are incentivizing the adoption of advanced energy storage technologies, spurring further investment in electrode materials innovation.
Looking ahead, the next few years are expected to see continued breakthroughs in material synthesis, scale-up, and cost reduction. Collaborative efforts between material suppliers, device manufacturers, and end-users are likely to yield commercially viable solutions that bridge the gap between laboratory performance and real-world deployment. As supercapacitor electrode materials engineering matures, the sector is poised for robust growth, with expanding opportunities in electric vehicles, smart grids, and industrial automation.
Global Market Size and Forecast (2025–2030): CAGR and Revenue Projections
The global market for supercapacitor electrode materials is poised for robust growth between 2025 and 2030, driven by accelerating demand for high-performance energy storage solutions in automotive, grid, and consumer electronics sectors. As of 2025, the market is estimated to be valued at approximately USD 1.2–1.5 billion, with a projected compound annual growth rate (CAGR) of 15–18% through 2030. This expansion is underpinned by rapid advancements in electrode material engineering, particularly in the development and commercialization of advanced carbon-based materials, metal oxides, and emerging hybrid composites.
Key industry players such as Kuraray Co., Ltd., a leading supplier of activated carbon materials, and Cabot Corporation, known for its conductive carbon additives, are scaling up production capacities to meet surging demand from electric vehicle (EV) manufacturers and grid storage integrators. Skeleton Technologies, a European innovator, is investing in next-generation graphene-based electrodes, aiming to deliver supercapacitors with higher energy densities and longer lifespans. These companies are actively expanding their global footprints, with new manufacturing facilities and strategic partnerships announced for 2025 and beyond.
The Asia-Pacific region, led by China, Japan, and South Korea, is expected to dominate both production and consumption of supercapacitor electrode materials. Major regional manufacturers such as Toray Industries, Inc. and Kyocera Corporation are investing in R&D to enhance the performance and cost-effectiveness of carbon nanomaterials and transition metal oxides. Meanwhile, North American and European markets are witnessing increased adoption in automotive and renewable energy storage applications, supported by government incentives and sustainability mandates.
Looking ahead, the market outlook remains highly positive, with revenue projections reaching USD 2.5–3.0 billion by 2030. This growth will be fueled by continued innovation in electrode material engineering, including the commercialization of novel materials such as doped graphene, carbon nanotubes, and hybrid organic-inorganic composites. Industry stakeholders anticipate that ongoing improvements in energy density, cycle life, and cost reduction will further accelerate the integration of supercapacitors across diverse sectors, solidifying their role in the global energy transition.
Material Innovations: Graphene, Carbon Nanotubes, and Beyond
The landscape of supercapacitor electrode materials engineering is undergoing rapid transformation, with a pronounced focus on advanced carbon-based materials such as graphene and carbon nanotubes (CNTs). These innovations are driven by the need for higher energy and power densities, longer cycle life, and improved scalability for commercial applications. As of 2025, several industry leaders and research-driven manufacturers are pushing the boundaries of what is possible in supercapacitor performance through material innovation.
Graphene, with its exceptional electrical conductivity, high surface area, and mechanical strength, remains at the forefront of supercapacitor electrode development. Companies like First Graphene Limited are actively commercializing high-purity graphene materials specifically tailored for energy storage applications, including supercapacitors. Their proprietary production processes aim to deliver consistent quality and scalability, addressing one of the key challenges in the field. Similarly, Directa Plus is supplying graphene-based products for energy storage, focusing on environmentally friendly production and integration into composite electrodes.
Carbon nanotubes are also gaining traction due to their unique one-dimensional structure, which facilitates rapid electron and ion transport. OCSiAl, recognized as one of the world’s largest single-wall carbon nanotube producers, is collaborating with supercapacitor manufacturers to enhance electrode conductivity and mechanical stability. Their materials are being integrated into next-generation devices to achieve higher capacitance and improved cycle life.
Beyond graphene and CNTs, hybrid materials and composites are emerging as promising candidates. Companies such as Arkema are developing advanced carbon materials and polymer composites that combine the advantages of different nanostructures, aiming to optimize both energy and power density. These hybrid electrodes often incorporate pseudocapacitive materials (e.g., metal oxides or conducting polymers) with carbon nanostructures to further boost performance.
Looking ahead, the next few years are expected to see increased commercialization of these advanced materials, with a focus on cost reduction, process scalability, and environmental sustainability. Industry collaborations and pilot-scale production lines are being established to bridge the gap between laboratory breakthroughs and mass-market adoption. The ongoing efforts by companies like First Graphene Limited, OCSiAl, and Arkema are likely to set new benchmarks in supercapacitor performance, paving the way for broader deployment in automotive, grid storage, and consumer electronics sectors.
Manufacturing Advances and Scalability Challenges
The engineering of supercapacitor electrode materials is undergoing rapid transformation in 2025, driven by the dual imperatives of manufacturing scalability and performance optimization. The sector is witnessing a shift from laboratory-scale innovations to industrial-scale production, with a focus on cost-effective, high-throughput processes for advanced materials such as graphene, carbon nanotubes, and transition metal oxides.
One of the most significant advances is the adoption of roll-to-roll manufacturing techniques for electrode fabrication. Companies like Maxwell Technologies (a subsidiary of Tesla) have been instrumental in scaling up the production of activated carbon-based electrodes, leveraging automated coating and calendaring processes to ensure uniformity and high yield. This approach is being mirrored by Asian manufacturers such as Panasonic Corporation and Skeleton Technologies, who are investing in pilot lines for graphene-enhanced electrodes, aiming to bridge the gap between laboratory performance and commercial viability.
Material purity and consistency remain critical challenges. The integration of advanced quality control systems, including in-line spectroscopy and machine vision, is becoming standard practice among leading producers. For instance, Skeleton Technologies has reported the deployment of proprietary quality assurance protocols to monitor the microstructure of their patented “curved graphene” materials, which are central to their high-power supercapacitor products.
Supply chain scalability is another focal point. The demand for sustainable and abundant raw materials is prompting companies to explore bio-derived carbons and recycled feedstocks. Panasonic Corporation has announced initiatives to incorporate biomass-derived activated carbons, aiming to reduce environmental impact and secure long-term supply. Meanwhile, Maxwell Technologies continues to refine its sourcing strategies for high-purity carbon precursors, balancing cost and performance.
Despite these advances, several scalability challenges persist. Uniform dispersion of nanomaterials, electrode thickness control, and binder compatibility are ongoing technical hurdles. The industry is also contending with the need for standardized testing protocols to ensure cross-comparability of performance metrics, a topic actively addressed by industry consortia and standards bodies.
Looking ahead, the next few years are expected to see further integration of automation, digital twins, and AI-driven process optimization in electrode manufacturing. The push towards gigafactory-scale production, as exemplified by Skeleton Technologies’s expansion plans, signals a maturing sector poised to meet the growing demand for high-performance, scalable supercapacitor solutions across automotive, grid, and industrial applications.
Performance Metrics: Energy Density, Power Density, and Lifespan
The performance of supercapacitors is fundamentally determined by the engineering of their electrode materials, with energy density, power density, and lifespan serving as the primary metrics of evaluation. As of 2025, the industry is witnessing rapid advancements in material science, driven by the need to bridge the gap between batteries and traditional capacitors.
Energy density, typically measured in Wh/kg, remains a focal point for supercapacitor development. Conventional activated carbon electrodes, while offering high surface area, are limited in energy density (usually below 10 Wh/kg). Recent years have seen a shift towards hybrid materials, such as graphene composites and transition metal oxides, which are being actively developed by leading manufacturers. For instance, Maxwell Technologies (now part of Tesla) and Skeleton Technologies are investing in graphene-based electrodes, reporting energy densities approaching 20 Wh/kg in commercial prototypes. These improvements are attributed to enhanced surface area, conductivity, and tailored pore structures that facilitate efficient ion transport.
Power density, measured in kW/kg, is another critical metric, with supercapacitors traditionally excelling in this area due to their rapid charge/discharge capabilities. State-of-the-art devices from Skeleton Technologies and Eaton routinely achieve power densities exceeding 10 kW/kg, enabled by low-resistance electrode materials and optimized cell architectures. The use of pseudocapacitive materials, such as manganese dioxide and conductive polymers, is being explored to further boost both energy and power densities, though challenges remain in balancing these properties with long-term stability.
Lifespan, often quantified in cycle life, is a key differentiator for supercapacitors compared to batteries. Modern devices can endure over one million charge/discharge cycles with minimal capacity fade, thanks to robust electrode materials and advanced electrolytes. Companies like CAP-XX and Eaton emphasize the reliability of their products for automotive and industrial applications, where longevity is paramount. Ongoing research focuses on mitigating degradation mechanisms, such as electrode corrosion and electrolyte decomposition, through surface engineering and the development of stable material interfaces.
Looking ahead, the next few years are expected to bring further improvements in all three performance metrics. The integration of nanostructured materials, scalable manufacturing techniques, and AI-driven material discovery are poised to accelerate progress. As supercapacitor electrode materials continue to evolve, the industry anticipates broader adoption in sectors demanding high power, fast charging, and long operational lifespans.
Key Players and Strategic Partnerships (e.g., Skeleton Technologies, Maxwell Technologies, Panasonic)
The supercapacitor sector is witnessing a dynamic phase in electrode materials engineering, with leading companies intensifying R&D and forging strategic partnerships to accelerate innovation. As of 2025, the focus is on advanced carbon materials, hybrid composites, and scalable manufacturing processes to meet the growing demand for high-performance energy storage in automotive, grid, and industrial applications.
Skeleton Technologies, a European frontrunner, continues to push the boundaries of supercapacitor performance through its proprietary “curved graphene” material. This technology, developed in-house, offers significantly higher energy and power densities compared to conventional activated carbon electrodes. In 2024–2025, Skeleton has expanded its manufacturing capacity in Germany and deepened collaborations with automotive OEMs and grid solution providers, aiming to commercialize next-generation modules for electric vehicles and heavy industry. The company’s partnerships with major players in the automotive and rail sectors underscore its commitment to integrating advanced electrode materials into real-world applications (Skeleton Technologies).
Maxwell Technologies, now a subsidiary of Tesla, Inc., remains a key innovator in supercapacitor electrode engineering. Maxwell’s legacy in dry electrode technology, which leverages advanced carbon materials, is being further developed under Tesla’s stewardship. The integration of Maxwell’s expertise into Tesla’s broader energy storage initiatives is expected to yield new electrode architectures with improved cycle life and energy density, targeting both automotive and stationary storage markets. The synergy between Maxwell’s supercapacitor know-how and Tesla’s battery manufacturing scale is anticipated to accelerate the commercialization of hybrid energy storage systems in the coming years (Maxwell Technologies).
Panasonic Corporation continues to invest in supercapacitor R&D, focusing on electrode material optimization and miniaturization for consumer electronics and industrial automation. Panasonic’s research centers in Japan are exploring novel carbon composites and hybrid materials to enhance capacitance and reduce internal resistance. The company’s strategic alliances with electronics manufacturers and component suppliers are aimed at integrating advanced supercapacitor modules into next-generation devices, with pilot projects underway in robotics and IoT infrastructure (Panasonic Corporation).
Looking ahead, the supercapacitor industry is expected to see further consolidation and cross-sector partnerships, particularly as automotive electrification and renewable integration drive demand for rapid-charging, long-life energy storage. The ongoing collaboration between material suppliers, device manufacturers, and end-users will be crucial in translating laboratory advances in electrode materials into scalable, cost-effective commercial products by 2026 and beyond.
Application Landscape: Automotive, Grid Storage, Consumer Electronics
Supercapacitor electrode materials engineering is rapidly shaping the application landscape across automotive, grid storage, and consumer electronics sectors as of 2025. The drive for higher energy density, faster charge/discharge rates, and longer cycle life is pushing manufacturers and material scientists to innovate beyond traditional activated carbon electrodes. In the automotive sector, the integration of supercapacitors is accelerating, particularly for start-stop systems, regenerative braking, and hybrid powertrains. Companies such as Maxwell Technologies (a subsidiary of Tesla) and Skeleton Technologies are at the forefront, leveraging advanced carbon-based materials and proprietary curved graphene to deliver modules with improved power density and operational lifespans suitable for commercial vehicles and buses.
In grid storage, the need for rapid response and high cycle stability is driving the adoption of supercapacitors for frequency regulation, voltage stabilization, and bridging power. Skeleton Technologies has deployed large-scale supercapacitor banks in European grid projects, utilizing their patented “curved graphene” electrodes to achieve higher capacitance and lower equivalent series resistance (ESR). Meanwhile, Eaton is integrating supercapacitor modules into uninterruptible power supply (UPS) systems and grid support solutions, focusing on reliability and safety for critical infrastructure.
Consumer electronics continue to benefit from miniaturized supercapacitor modules, with companies like Panasonic and Murata Manufacturing advancing the use of high-surface-area carbon and hybrid electrode materials. These innovations enable rapid charging and discharging in devices such as wearables, wireless sensors, and backup power units. The trend toward flexible and solid-state supercapacitors is also emerging, with research and pilot production focusing on polymer composites and metal oxide nanostructures to further enhance energy density and mechanical flexibility.
Looking ahead to the next few years, the sector is expected to see increased commercialization of hybrid electrode materials—combining carbon with transition metal oxides or conducting polymers—to bridge the gap between supercapacitors and batteries. The automotive and grid sectors are likely to benefit most from these advances, as manufacturers seek to meet stricter energy efficiency and sustainability targets. Strategic partnerships between material suppliers, such as 3M, and supercapacitor manufacturers are anticipated to accelerate the scale-up of novel electrode technologies, supporting broader adoption across all major application domains.
Sustainability and Environmental Impact of Electrode Materials
The sustainability and environmental impact of supercapacitor electrode materials are increasingly central to both research and commercial strategies as the sector matures in 2025. Traditional electrode materials, such as activated carbon derived from non-renewable sources, are being scrutinized for their lifecycle emissions and end-of-life disposal challenges. In response, leading manufacturers and research institutions are accelerating the development of greener alternatives, including bio-derived carbons, transition metal oxides, and conductive polymers.
A notable trend is the adoption of biomass-derived carbons, which utilize agricultural waste or other renewable feedstocks. Companies such as Norit, a major producer of activated carbon, are exploring sustainable sourcing and processing methods to reduce the carbon footprint of their products. Similarly, Cabot Corporation is investing in the development of low-emission carbon materials, with a focus on circular economy principles and closed-loop manufacturing.
The environmental impact of transition metal oxides, such as manganese oxide and nickel cobaltite, is also under review. While these materials offer high capacitance, their extraction and processing can be energy-intensive and associated with toxic byproducts. To address this, companies like Umicore are implementing responsible sourcing and recycling initiatives, aiming to minimize the ecological footprint of metal-based electrode materials.
Conductive polymers, such as polyaniline and polypyrrole, are gaining traction due to their tunable properties and potential for lower environmental impact. However, the sustainability of their synthesis routes remains a challenge. Industry players are collaborating with academic partners to develop greener polymerization processes and to assess the biodegradability of these materials.
End-of-life management is another critical aspect. The recyclability of supercapacitor electrodes is being prioritized, with companies like Maxwell Technologies (a subsidiary of Tesla) and Skeleton Technologies exploring closed-loop recycling systems and second-life applications for spent devices. These efforts are supported by evolving regulatory frameworks in the EU and Asia, which are expected to tighten requirements for material recovery and reporting in the coming years.
Looking ahead, the sector is poised for further advances in green chemistry, circular design, and supply chain transparency. The integration of life cycle assessment (LCA) tools into product development is becoming standard practice, enabling manufacturers to quantify and reduce the environmental impact of their electrode materials. As sustainability becomes a key differentiator, companies that can demonstrate low-impact, high-performance solutions are likely to gain a competitive edge in the global supercapacitor market.
Regulatory Standards and Industry Initiatives (e.g., ieee.org, sae.org)
The regulatory landscape and industry initiatives surrounding supercapacitor electrode materials engineering are rapidly evolving as the technology matures and finds broader applications in transportation, grid storage, and consumer electronics. In 2025, the focus is on harmonizing safety, performance, and sustainability standards to support the commercialization and integration of advanced supercapacitor systems.
Key industry bodies such as the IEEE and the SAE International are at the forefront of developing and updating standards relevant to supercapacitor technologies. The IEEE has established standards like IEEE 1679.1, which provides guidelines for the characterization and evaluation of electric double-layer capacitors (EDLCs), including those with novel electrode materials such as graphene, carbon nanotubes, and transition metal oxides. These standards are being revised to address the latest advancements in electrode engineering, including the integration of nanostructured materials and hybrid composites, which are expected to dominate the market in the coming years.
SAE International is also actively engaged in standardizing testing protocols and safety requirements for supercapacitors used in automotive and aerospace applications. The SAE J3078 series, for example, outlines performance and safety testing for ultracapacitors, with recent updates reflecting the increased energy densities and new chemistries enabled by advanced electrode materials. These standards are critical as automakers and aerospace manufacturers, such as Tesla, Inc. and Airbus, explore the integration of supercapacitors for high-power, fast-charging, and regenerative braking systems.
On the industry initiative front, leading supercapacitor manufacturers like Maxwell Technologies (a subsidiary of Tesla), Skeleton Technologies, and Eaton are collaborating with standards organizations to ensure that new electrode materials meet both regulatory and market requirements. These companies are investing in R&D to develop electrodes with higher conductivity, longer cycle life, and improved environmental profiles, while also participating in working groups to shape future standards.
Looking ahead, regulatory attention is expected to intensify around the sourcing and recyclability of electrode materials, particularly as the use of rare or potentially hazardous substances increases. Industry consortia and alliances are forming to address lifecycle management and to promote the adoption of green manufacturing practices. The next few years will likely see the introduction of stricter guidelines for material traceability, environmental impact, and end-of-life handling, aligning with global sustainability goals and the circular economy.
Future Outlook: Disruptive Technologies and Market Opportunities
The landscape of supercapacitor electrode materials engineering is poised for significant transformation in 2025 and the following years, driven by both technological breakthroughs and evolving market demands. The push for higher energy density, faster charging, and longer cycle life is accelerating the adoption of advanced materials and hybrid architectures, with a strong focus on sustainability and cost-effectiveness.
A key area of innovation is the development of next-generation carbon-based electrodes. Companies such as Nippon Carbon and Kuraray are advancing the use of activated carbon, carbon nanotubes, and graphene derivatives to enhance surface area and conductivity. These materials are being engineered at the nanoscale to optimize pore structure, enabling higher capacitance and improved charge/discharge rates. In parallel, Skeleton Technologies is commercializing curved graphene materials, which have demonstrated significant improvements in power density and operational lifespan, positioning them as a disruptive force in sectors such as automotive and grid storage.
Transition metal oxides and conducting polymers are also gaining traction as electrode materials, offering the potential for hybrid supercapacitors that bridge the gap between traditional capacitors and batteries. Companies like Maxwell Technologies (now part of Tesla) are exploring manganese oxide and other pseudocapacitive materials to achieve higher energy densities. The integration of these materials with carbon-based substrates is expected to yield devices with both high power and energy capabilities, suitable for applications ranging from regenerative braking to renewable energy buffering.
Sustainability is becoming a central theme in materials engineering. Efforts are underway to utilize bio-derived carbons and recycled materials, reducing environmental impact and aligning with global decarbonization goals. Kuraray and other suppliers are investing in green chemistry approaches and closed-loop manufacturing processes, anticipating regulatory shifts and consumer preferences for eco-friendly energy storage solutions.
Looking ahead, the market for supercapacitor electrode materials is expected to expand rapidly, driven by electrification trends in transportation, industrial automation, and smart grid infrastructure. Strategic partnerships between material suppliers, device manufacturers, and end-users are likely to accelerate commercialization cycles. As performance benchmarks continue to rise, the sector is set for disruptive growth, with advanced electrode engineering at the core of next-generation supercapacitor technologies.