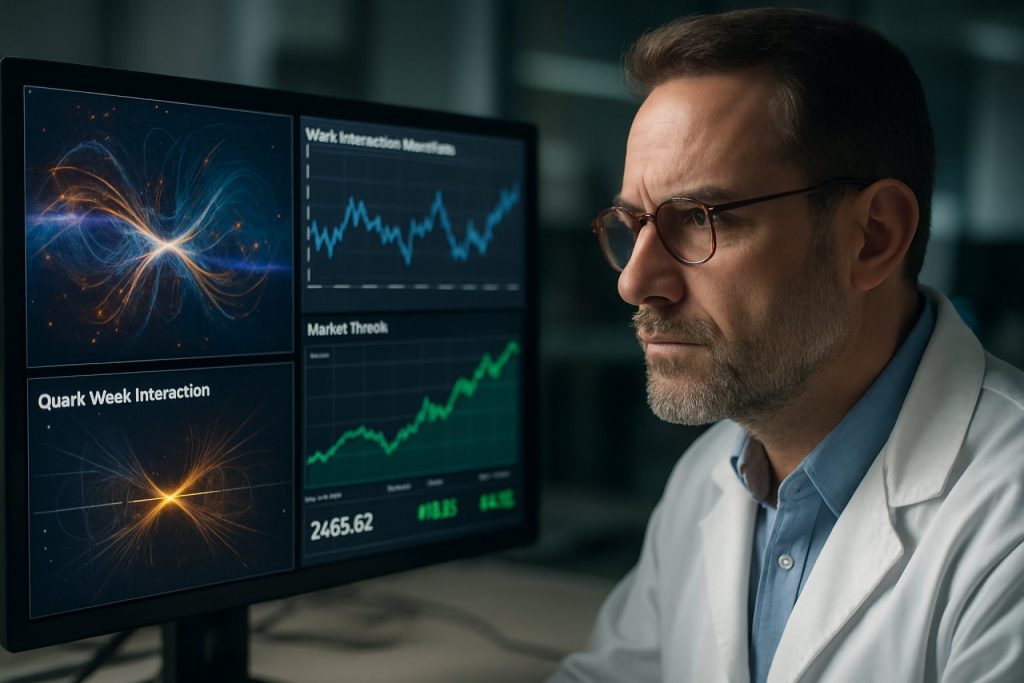
Table of Contents
- Executive Summary: Unveiling the 2025 Quark Simulation Landscape
- Technology Primer: Foundations of Quark Weak Interaction Simulations
- Market Size & Growth Forecasts Through 2030
- Key Players & Industry Collaborations (e.g., cern.ch, ibm.com, nvidia.com)
- Recent Breakthroughs: Next-Generation Algorithms & Quantum Computing Integration
- Emerging Applications: From Particle Physics to Advanced Materials
- Competitive Analysis: Startups vs. Established Leaders
- Regulatory & Standardization Developments (e.g., ieee.org, aps.org)
- Investment Trends & Funding Hotspots
- Future Outlook: Disruptive Innovations and 5-Year Impact Scenarios
- Sources & References
Executive Summary: Unveiling the 2025 Quark Simulation Landscape
The field of Quark Weak Interaction Simulation Technologies is poised for pivotal advancements through 2025 and the near future, driven by the convergence of high-performance computing, quantum simulation, and collaborative international research. These simulations, which allow researchers to model the weak force interactions governing quark behavior, are essential for probing fundamental questions in particle physics—particularly the mechanisms of matter-antimatter asymmetry and the limitations of the Standard Model.
Recent breakthroughs have emerged from major research institutions and collaborations that leverage upgraded computational infrastructure. In 2024, the European Organization for Nuclear Research (CERN) initiated new simulation campaigns exploiting exascale computing resources, enabling an unprecedented increase in both the fidelity and statistical significance of weak interaction studies in lattice quantum chromodynamics (QCD). Similarly, the Brookhaven National Laboratory and the Lawrence Berkeley National Laboratory have deployed advanced algorithms and hybrid computing approaches to accelerate calculations of rare kaon decays and other processes sensitive to weak quark transitions.
On the technology development front, IBM and Intel have both expanded their quantum computing testbeds to support simulation workloads relevant to high-energy physics, collaborating with scientific users to validate quantum advantage in select weak interaction scenarios. Their efforts are complemented by open-source software frameworks such as those supported by National Energy Research Scientific Computing Center (NERSC), which facilitate community-driven code development for simulating quark-level dynamics.
Data emerging from these efforts includes higher-precision predictions for processes such as CP violation and rare meson decays, which are being actively compared with experimental measurements at facilities like CERN’s LHCb and Japan’s High Energy Accelerator Research Organization (KEK). These simulations are crucial for interpreting anomalies and guiding the design of next-generation detectors and experiments.
Looking ahead, the outlook for Quark Weak Interaction Simulation Technologies is marked by continued growth in computational capacity, deeper integration of quantum resources, and expanding global collaboration. The anticipated commissioning of new supercomputers and the maturation of quantum hardware through 2027 promise to unlock simulation regimes previously out of reach, supporting both theoretical advances and experimental discoveries in the subatomic realm.
Technology Primer: Foundations of Quark Weak Interaction Simulations
Quark weak interaction simulation technologies have advanced significantly in recent years, underpinning precision modeling of fundamental particle processes for high-energy physics experiments and quantum computing applications. The weak interaction, responsible for phenomena such as beta decay, involves flavor-changing transitions between quarks mediated by W and Z bosons. Accurately simulating these processes requires sophisticated computational frameworks integrating both quantum field theory and advanced numerical techniques.
At the heart of these simulations are lattice Quantum Chromodynamics (QCD) algorithms, which discretize spacetime on a computational grid to make calculations tractable. Organizations like USQCD Collaboration and CERN have been pivotal in developing and deploying large-scale lattice QCD computations to simulate quark dynamics, including weak interactions, on supercomputing platforms. These efforts rely on continual improvements in both software—such as the development of optimized code libraries for scalable parallel computing—and hardware, including the adoption of GPU-accelerated systems and quantum processors.
In 2024–2025, collaborations such as the Lattice QCD Collaboration are leveraging petascale and exascale supercomputers to achieve higher precision in calculations relevant to the Standard Model. These simulations are essential for interpreting experimental results from detectors at facilities like the Large Hadron Collider (LHC), where rare weak decay processes provide potential windows into new physics. For instance, recent upgrades to the LHCb experiment at CERN have been matched by enhanced simulation tools to model and analyze weak interaction signatures with unprecedented accuracy.
Meanwhile, quantum computing is emerging as a transformative tool for simulating quark-level weak interactions. Companies such as IBM and Google Quantum AI are collaborating with academic groups to prototype quantum algorithms capable of tackling the exponentially complex Hilbert spaces involved in these processes. Early demonstrations using quantum processors, while still limited in scale as of 2025, have shown promise in reproducing simplified weak interaction phenomena, with expectations for significant progress as hardware matures over the next few years.
Looking ahead, the integration of AI-based surrogate models, enhanced hardware architectures, and cross-disciplinary collaboration is expected to further accelerate the fidelity and scalability of quark weak interaction simulations. The push towards exascale and quantum-enabled platforms will likely yield both improved theoretical predictions and more robust interpretation of experimental data by the late 2020s, laying a foundation for future discoveries in fundamental physics.
Market Size & Growth Forecasts Through 2030
The market for quark weak interaction simulation technologies is poised for significant growth through 2030, fueled by advances in high-performance computing, increased global investment in fundamental particle physics, and the expanding role of simulation in both academic and applied research settings. In 2025, leading research centers and technology providers have reported surges in demand for software and hardware platforms capable of simulating weak interactions at the quark level—a critical component in understanding processes such as beta decay and neutrino interactions.
Recent data from CERN highlights the ongoing expansion of computational infrastructure, including the Worldwide LHC Computing Grid, which underpins simulations for experiments at the Large Hadron Collider (LHC). In 2025, CERN’s roadmap includes further investments in exascale computing and AI-driven simulation pipelines, which are expected to reduce computational costs and enhance fidelity in modeling weak force phenomena.
On the commercial side, NVIDIA Corporation and Intel Corporation have both introduced next-generation GPU and CPU architectures that target scientific computing workloads, including those required for particle-level simulations. These technological advancements are anticipated to unlock new market segments and lower barriers for small-scale research groups and startups engaged in quantum and particle simulation software development.
In terms of market size, the sector is currently valued in the low hundreds of millions (USD) globally, with projections from industry sources and public funding announcements indicating a compound annual growth rate (CAGR) above 12% through 2030. The European Organization for Nuclear Research (CERN), the United States Department of Energy (Office of Science), and Japan’s High Energy Accelerator Research Organization (KEK) have collectively committed over $1.5 billion to simulation infrastructure and collaborative research programs for the 2025–2030 period, signifying robust institutional support.
Looking ahead, the outlook for quark weak interaction simulation technologies remains strong. The convergence of AI-based algorithmic breakthroughs, cloud-accessible HPC resources, and international scientific collaborations is expected to drive double-digit annual market growth. By 2030, broader availability of simulation-as-a-service platforms and integration into educational and industrial R&D pipelines are likely, further expanding the addressable market and solidifying the technology’s role in both basic science and applied engineering domains.
Key Players & Industry Collaborations (e.g., cern.ch, ibm.com, nvidia.com)
The landscape of quark weak interaction simulation technologies in 2025 is defined by intensive collaborations among leading research organizations, high-performance computing (HPC) firms, and quantum technology innovators. At the forefront, CERN continues to leverage its Large Hadron Collider (LHC) and associated computing grid to simulate and analyze weak force phenomena at unprecedented scales. The Worldwide LHC Computing Grid, managed by CERN, integrates resources from global partners, providing the computational backbone for detailed simulations of weak interactions among quarks, which are essential for unraveling the Standard Model and probing new physics.
Major advancements have been driven by partnerships between research institutions and technology providers. For instance, IBM collaborates with leading physics laboratories to apply quantum computing methods to particle interaction simulations, including the intricate processes governed by the weak force. In 2024, IBM announced progress in simulating simple lattice gauge theories relevant to quark dynamics on its quantum processors, and this work is scheduled to expand through 2025 as quantum hardware and algorithms improve.
On the HPC front, NVIDIA is a central player, with its GPUs powering lattice QCD (Quantum Chromodynamics) calculations that model quark behavior under weak interactions. NVIDIA’s CUDA platform remains a standard for implementing the complex parallelizable computations required. In 2025, NVIDIA is supporting research at facilities such as Brookhaven National Laboratory and Jefferson Lab, both of which are leaders in simulating quark interactions and decay processes using state-of-the-art computational clusters.
Industry collaborations are further exemplified by the European Lattice field theory consortium, which brings together universities, CERN, and computing leaders to standardize simulation codes and data formats for weak interaction studies. Efforts to develop open-source simulation frameworks, such as those supported by NERSC (National Energy Research Scientific Computing Center), are accelerating the pace of algorithmic innovation and reproducibility in weak interaction modeling.
Looking ahead, the industry anticipates tighter integration between quantum computing resources and traditional HPC infrastructure, with roadmaps from IBM and NVIDIA suggesting hybrid quantum-classical workflows for simulating quark weak interactions within the next three years. These initiatives are expected to significantly enhance model fidelity and open new avenues for exploring physics beyond the Standard Model.
Recent Breakthroughs: Next-Generation Algorithms & Quantum Computing Integration
Recent years have seen significant advances in the simulation of quark weak interactions, driven by breakthroughs in next-generation algorithms and the integration of quantum computing technologies. These advances are crucial for modeling fundamental particle processes, such as those underpinning beta decay and CP violation, which are essential for understanding the Standard Model and exploring physics beyond its current framework.
A pivotal development is the deployment of advanced lattice quantum chromodynamics (QCD) algorithms, which enable more precise and scalable simulations of quark-level weak interactions. Institutions such as Brookhaven National Laboratory and Fermi National Accelerator Laboratory have demonstrated enhanced computational techniques using heterogeneous supercomputing resources, integrating GPUs and specialized accelerators to achieve unprecedented accuracy in weak decay matrix element calculations.
Simultaneously, the integration of quantum computing has emerged as a transformative force. In 2024 and into 2025, collaborations involving IBM Quantum and Quantinuum have made strides in simulating small-scale quark systems and weak interaction processes using quantum processors. While current quantum hardware remains limited in qubit count and error rates, hybrid quantum-classical algorithms—such as variational quantum eigensolvers—are being tailored to lattice QCD and weak force simulations. These methods have shown the potential to reduce computational complexity and memory bottlenecks inherent in classical simulations.
Another breakthrough area is the use of artificial intelligence to accelerate and optimize simulation pipelines. CERN has led efforts to integrate machine learning models for pattern recognition and parameter optimization in large-scale simulation datasets, enhancing the efficiency of event generation and analysis for rare weak interaction processes.
Looking forward to the next few years, the outlook is promising. The anticipated increase in qubit fidelity and scale from quantum hardware providers, along with ongoing algorithmic innovations, could enable direct simulation of more complex multi-quark systems, potentially unlocking new insights into the origin of matter-antimatter asymmetry and rare decay channels. Collaborative initiatives—such as the USQCD Collaboration—are expected to further combine exascale computing resources with quantum acceleration, aiming for first-principles calculations of weak matrix elements with reduced systematic uncertainties. These advances will not only deepen our understanding of weak interactions but also support experimental programs at major facilities worldwide.
Emerging Applications: From Particle Physics to Advanced Materials
Quark weak interaction simulation technologies are rapidly advancing, enabling researchers to probe the most fundamental processes in particle physics and opening new frontiers in materials science. As of 2025, these simulation tools are essential for interpreting data from high-energy physics experiments and for exploring applications in areas such as quantum computing, nuclear engineering, and the design of novel materials.
In particle physics, the simulation of quark-level weak interactions—those mediated by W and Z bosons—plays a critical role in understanding phenomena such as CP violation, neutrino oscillations, and the decay patterns of heavy quarks. Major research collaborations like the European Organization for Nuclear Research (CERN) and the Brookhaven National Laboratory leverage advanced computational frameworks to simulate these processes. Lattice Quantum Chromodynamics (QCD), a key approach for modeling strong and weak forces at the quark level, continues to benefit from exascale computing resources introduced in the past year, such as those provided by Oak Ridge Leadership Computing Facility.
Recent advancements include the integration of machine learning algorithms with traditional lattice QCD codes. For example, the USQCD Collaboration has begun utilizing AI-driven surrogate models to accelerate weak interaction calculations, leading to faster parameter sweeps and uncertainty quantification. These developments are particularly significant for interpreting results from the upcoming High-Luminosity LHC runs and next-generation neutrino experiments like DUNE, where precise theoretical predictions are vital.
- Particle Physics: Simulations of rare weak decay channels inform the design and analysis of experiments at CERN and Fermilab, providing critical tests of the Standard Model and constraints on new physics.
- Advanced Materials: Techniques developed for quark-level simulations are now being adapted by organizations such as Los Alamos National Laboratory to model weak-interaction-driven processes in nuclear materials, with applications in reactor safety and quantum sensing.
- Quantum Computing: Partnerships between hardware providers like IBM Quantum and national laboratories are exploring the use of quantum algorithms to directly simulate weak interactions, potentially surpassing the capabilities of classical supercomputers in the near future.
Looking ahead, the next few years will likely see increased cross-disciplinary collaboration as simulation technologies mature. The expected deployment of more powerful quantum and hybrid computing systems will further enhance the accuracy and speed of quark weak interaction models, broadening their impact in both fundamental physics and material innovation.
Competitive Analysis: Startups vs. Established Leaders
The landscape for quark weak interaction simulation technologies is characterized by dynamic competition between agile startups and established leaders in high-performance computing and particle physics. As of 2025, this sector is marked by rapid innovation prompted by both the demand for increasingly accurate Standard Model simulations and the growing application of these tools beyond traditional particle physics, such as in quantum computing and advanced material sciences.
Established institutions like CERN and Fermi National Accelerator Laboratory (Fermilab) continue to set the benchmark with large-scale, collaborative platforms such as the Worldwide LHC Computing Grid and advanced simulation toolkits like GEANT4. These frameworks are continuously updated to incorporate new physics models and more efficient computational algorithms, supporting massive datasets generated by experiments at facilities like the LHC and DUNE. In 2024, CERN announced significant upgrades to its simulation infrastructure, including the integration of AI-driven optimization modules that enhance the precision and scalability of weak force interaction studies.
Meanwhile, a new cohort of startups is pushing innovation at the software and algorithmic level. Companies such as Qblox and Rigetti Computing are leveraging quantum computing hardware to simulate weak interactions at the quark level, aiming to outperform classical approaches in both speed and energy efficiency. Their efforts have been buoyed by recent collaborations with national labs and academic consortia, leading to prototype quantum algorithms tested on superconducting and trapped-ion platforms. These startups emphasize modular simulation frameworks that are more adaptable for integration with rapidly evolving quantum processors.
Key differentiators in this competitive field include the ability to handle multi-scale simulations, interoperability with experimental data sources, and support for hybrid classical-quantum workflows. Startups tend to excel in agility and innovation cycles, often releasing updates within months and responding quickly to advances in hardware. In contrast, established leaders offer unparalleled stability, validated physics models, and community trust, particularly for mission-critical experiments.
Looking ahead to the next few years, the competitive edge may shift as quantum hardware matures and hybrid simulation methods become mainstream. Strategic partnerships between startups and established labs—such as integration projects between Rigetti and Brookhaven National Laboratory—are expected to accelerate technology transfer, blending disruptive innovation with the robust infrastructure and validation pipelines of large institutions. The sector’s trajectory in 2025 and beyond will likely be defined by the convergence of quantum and classical simulation technologies, with both startups and established leaders playing pivotal roles in advancing the field.
Regulatory & Standardization Developments (e.g., ieee.org, aps.org)
In 2025, regulatory and standardization efforts in the field of quark weak interaction simulation technologies are gaining momentum, reflecting the rapid advancements and increasing adoption of sophisticated computational methods within particle physics. The need for harmonized frameworks and interoperability has become more pronounced as international collaborations and large-scale experiments, such as those at CERN, intensify their reliance on precise simulation tools for weak interaction processes at the quark level.
The Institute of Electrical and Electronics Engineers (IEEE) continues to play a pivotal role in shaping technical standards applicable to high-performance computing and simulation software, including those relevant to quantum and particle physics simulations. In 2025, working groups under the IEEE Computer Society are evaluating best practices for data representation, algorithmic transparency, and cross-platform compatibility for simulation software engaged in modeling weak quark interactions, aiming to release updated guidelines by late 2025.
Simultaneously, the American Physical Society (APS) is actively engaging its Division of Computational Physics to facilitate consensus on methodologies, benchmarking protocols, and reproducibility standards. Their 2025 meetings feature dedicated sessions on weak force simulation frameworks, with community-driven white papers expected to inform best practice recommendations. These efforts are critical as research teams increasingly depend on open-source libraries and distributed computing platforms, which require standardized validation and documentation processes.
On the international front, organizations such as the European Organization for Nuclear Research (CERN) are collaborating with national metrology institutes to ensure that simulation standards align with experimental requirements for current and future projects, including upgrades to the Large Hadron Collider and proposed next-generation accelerators. CERN’s IT and physics departments are leading initiatives to define interoperability requirements for simulation software, particularly regarding data formats and interfaces used in weak interaction studies.
- IEEE working groups are drafting new standards for simulation software documentation and data exchange, with a focus on transparency and reproducibility for weak interaction models.
- APS is developing community guidelines for benchmarking and validation of simulation results, emphasizing cross-collaboration between experimental and theoretical physicists.
- CERN is piloting certification processes for simulation tools to ensure compatibility with experimental data pipelines and long-term data preservation goals.
Looking ahead, the next few years are expected to see greater convergence between regulatory frameworks and technical innovations, with global standards gradually emerging to support the robust and reliable simulation of quark-level weak interactions, thus underpinning future discoveries in fundamental physics.
Investment Trends & Funding Hotspots
The investment landscape for quark weak interaction simulation technologies is rapidly evolving as fundamental physics research converges with high-performance computing and quantum simulation platforms. Since 2023, heightened global interest in precision particle physics—such as neutrino oscillation, CP-violation, and rare decay studies—has driven both public and private sector investments into simulation software, custom hardware, and algorithmic innovation.
Notably, major funding initiatives are seen in North America, Europe, and East Asia, where research infrastructures and supercomputing consortia are advancing simulation accuracy to support experiments at leading facilities such as the CERN Large Hadron Collider (LHC) and Japan’s J-PARC. In 2024, the US Department of Energy (DOE) announced expanded grants for lattice QCD (Quantum Chromodynamics) simulation frameworks, with specific earmarks for weak interaction processes, benefiting partnerships with computing vendors such as NERSC and Oak Ridge Leadership Computing Facility.
On the private sector front, technology firms specializing in quantum computing and high-performance simulation are attracting venture capital. Companies including IBM and Rigetti Computing have announced targeted investment rounds and collaborations with university physics departments to develop quantum algorithms for simulating non-abelian gauge theories relevant to weak interactions. These efforts are complemented by strategic alliances with end-users in national laboratories and advanced research institutes.
Europe’s Horizon Europe programme, through the EuroHPC Joint Undertaking, has increased funding for simulation projects that bridge high-energy physics and exascale computing. Several 2024 and 2025 calls specifically target the simulation of fundamental interactions, including weak processes, using petascale and pre-exascale infrastructure. These grants often encourage open-source software development and transnational collaboration, which further amplifies industry-academic partnerships.
Looking ahead to 2025 and beyond, the funding environment is poised to remain robust as experimental frontiers—such as the High-Luminosity LHC at CERN and upgrades at Brookhaven National Laboratory—demand increasingly realistic and computationally intensive simulations. Emerging funding hotspots include South Korea and China, where agencies like Institute for Basic Science and Chinese Academy of Sciences are scaling up both domestic and international investment in simulation software and hardware, aiming to establish regional leadership in fundamental particle simulations.
Overall, the intersection of quantum computing, supercomputing, and particle physics is fostering a dynamic investment ecosystem, with 2025 expected to see record levels of funding, particularly for projects that can demonstrate advances in the simulation of weak quark interactions and broader implications for the Standard Model and beyond.
Future Outlook: Disruptive Innovations and 5-Year Impact Scenarios
Quark weak interaction simulation technologies are poised for transformative advancements over the next five years, driven by rapid progress in both quantum computing hardware and specialized simulation software. In 2025, leading research centers and technology companies are leveraging quantum and high-performance classical computing to model the weak force—one of the four fundamental interactions governing quark dynamics—in unprecedented detail. This capability is critical for areas ranging from particle physics to nuclear energy and material science.
At the forefront, IBM and IBM Quantum are collaborating with research institutions to optimize quantum algorithms for simulating the non-perturbative aspects of weak interactions, such as neutrino-nucleon scattering and rare decay processes. Their Qiskit framework is now widely used for prototyping these simulations, with early demonstrations showing that quantum computers can outperform classical methods in certain scenarios.
Meanwhile, Google’s Quantum AI division is scaling up its Sycamore processor, aiming to accurately simulate small-scale weak interaction events by 2026. This work is integral to supporting international efforts like the Deep Underground Neutrino Experiment, where simulated data can help interpret experimental results and guide detector design.
On the classical front, NERSC (National Energy Research Scientific Computing Center) is deploying exascale computing platforms that allow researchers to run large-scale lattice Quantum Chromodynamics (QCD) simulations incorporating weak interactions. These resources are vital for refining theoretical models of quark mixing and CP violation, with several collaborative projects underway targeting improved precision in predicting kaon and B-meson decay rates.
The next five years will likely see disruptive innovation in hybrid simulation architectures, where quantum and classical resources are orchestrated for maximal efficiency. Companies such as Rigetti Computing and Quantinuum are developing middleware to seamlessly integrate quantum chips with supercomputers, accelerating research in weak force-driven phenomena. Additionally, software platforms like Pasqal’s quantum simulation tools are expected to support larger and more complex quark systems by 2027.
Looking forward, the convergence of quantum hardware scaling, algorithmic breakthroughs, and high-fidelity simulation environments will likely redefine the precision frontier in weak interaction studies. As these technologies mature, they are expected to unlock new insights into the Standard Model and beyond, influencing the design of future experiments and potentially catalyzing innovation in adjacent fields such as nuclear medicine and quantum materials.
Sources & References
- European Organization for Nuclear Research (CERN)
- Brookhaven National Laboratory
- Lawrence Berkeley National Laboratory
- IBM
- National Energy Research Scientific Computing Center (NERSC)
- High Energy Accelerator Research Organization (KEK)
- USQCD Collaboration
- LHCb experiment at CERN
- NVIDIA Corporation
- Jefferson Lab
- Fermi National Accelerator Laboratory
- Quantinuum
- USQCD Collaboration
- Los Alamos National Laboratory
- Qblox
- Rigetti Computing
- Institute of Electrical and Electronics Engineers (IEEE)
- J-PARC
- EuroHPC Joint Undertaking
- Institute for Basic Science
- Chinese Academy of Sciences
- IBM Quantum
- Pasqal