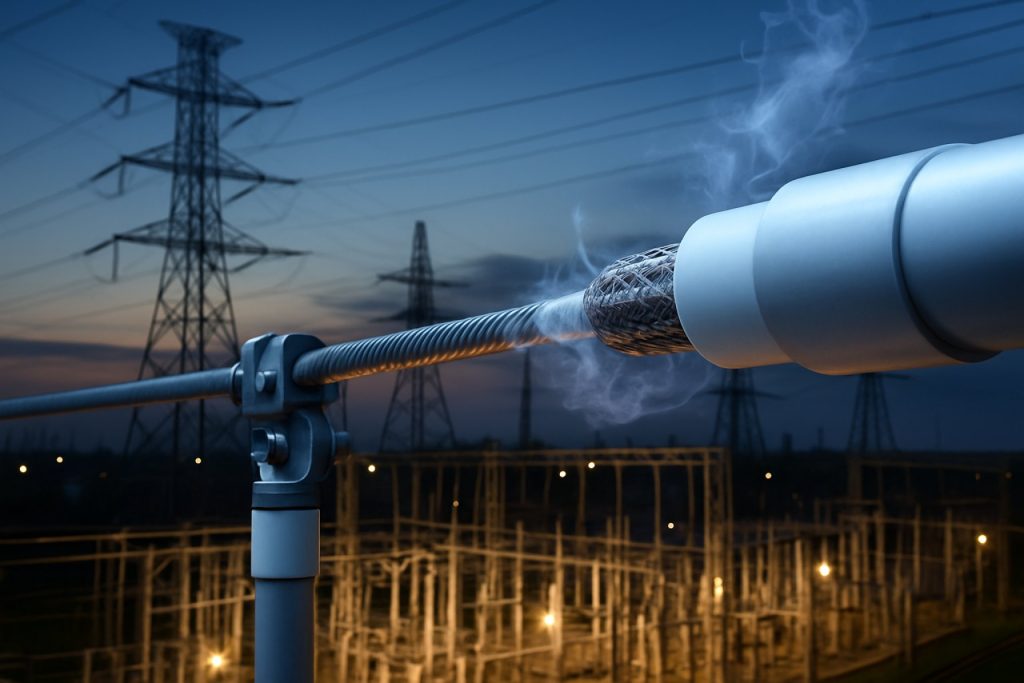
Table of Contents
- Executive Summary: The Future of Joule-Loss Balancing in Superconducting Power Grids
- Market Overview 2025: Trends, Drivers, and Barriers
- Technology Deep Dive: Mechanisms of Joule-Loss in Superconductors
- Key Players and Recent Innovations (2025)
- Economic Impact: Cost-Benefit Analysis for Utilities and Grid Operators
- Case Studies: Real-World Implementations and Pilot Projects
- Regulatory Landscape and Standards (IEEE, IEC, etc.)
- Market Forecasts: Growth Projections to 2030
- Emerging Technologies: AI, Sensors, and Advanced Materials
- Future Outlook: Opportunities, Challenges, and Strategic Recommendations
- Sources & References
Executive Summary: The Future of Joule-Loss Balancing in Superconducting Power Grids
Joule-loss balancing stands at the forefront of innovation in the development and deployment of superconducting power grids, promising a transformative impact on energy transmission as the world accelerates toward decarbonization and grid modernization in 2025 and beyond. Unlike conventional copper-based systems, superconducting cables offer near-zero resistance under cryogenic conditions, dramatically reducing ohmic losses, or Joule heating, in high-capacity power applications. However, practical deployment faces ongoing challenges related to energy dissipation at joints, connectors, and during transient fault conditions—issues that require sophisticated balancing strategies to ensure operational reliability and efficiency.
Recent demonstration projects and pilot installations underscore the momentum in this sector. For instance, Nexans has spearheaded several grid-scale superconducting cable deployments in Europe and Asia, integrating advanced monitoring systems to track and manage localized loss phenomena. Their work in Germany’s AmpaCity project and upcoming initiatives in China exemplify how real-time diagnostics and improved cable architectures are actively minimizing residual losses. Similarly, Sumitomo Electric Industries, Ltd. is advancing high-temperature superconducting (HTS) cable technology, focusing on joint engineering and system integration to mitigate energy leaks at interconnections—a primary contributor to non-ideal Joule losses.
Data from these projects indicate that, while superconducting cables can reduce total transmission losses by over 90% compared to traditional lines, loss balancing at terminations and in the presence of alternating current (AC) remains a technical hurdle. The SuperPower Inc. HTS cable systems, for example, have demonstrated stable operation in utility environments, yet ongoing refinements are needed to address AC loss components and ensure scalable deployment for urban grids.
Looking ahead to the next several years, industry experts anticipate that continued advances in cryogenic efficiency, joint design, and smart system controls will be pivotal for commercial-scale adoption. Organizations such as European Superconductivity Industry Association (ESIA) are coordinating research and standardization efforts to streamline loss balancing methodologies and accelerate market readiness. As governments and utilities prioritize resilient, low-loss transmission infrastructure, investments in superconducting technology are expected to grow, with pilot grids in Asia, Europe, and North America serving as critical testbeds for large-scale Joule-loss balancing solutions.
In summary, the next few years are poised to witness significant progress in the reduction and management of Joule losses in superconducting power grids, supported by robust cross-sector collaboration and a maturing ecosystem of manufacturers and utilities focused on grid innovation and sustainability.
Market Overview 2025: Trends, Drivers, and Barriers
Joule-loss balancing is emerging as a pivotal factor in the evolution of superconducting power grids, especially as grid operators and utilities seek to enhance energy efficiency and reliability. In traditional power transmission, resistive losses—termed “Joule losses”—account for a significant portion of energy inefficiency. Superconducting cables, by contrast, can carry much higher current densities with negligible resistive losses when cooled below their critical temperatures. As the industry moves into 2025, balancing the residual losses associated with superconducting systems—due to joints, connectors, and occasional local heating—remains a priority for ensuring grid stability and operational cost-effectiveness.
Recent project deployments and pilot networks illustrate the momentum behind superconducting technologies. For example, the Nexans ‘AmpaCity’ project in Essen, Germany continues to provide valuable operational data on loss management in urban superconducting cable applications. Similarly, Sumitomo Electric Industries and NKT are expanding their superconducting cable portfolios, focusing on optimizing cooling cycles and joint engineering to minimize localized Joule losses. These advancements are crucial as grid operators in Europe, Asia, and North America consider scaling up high-capacity superconducting links for dense urban and renewable-heavy regions.
Drivers for market growth in 2025 include intensifying grid decarbonization mandates, urbanization, and increased demand for reliable, high-capacity transmission. The integration of distributed renewable resources—increasingly volatile in output—necessitates low-loss transmission and rapid fault response, both of which superconducting grids can deliver. Further, governmental support in regions like the EU and Japan is accelerating demonstrations and standardization efforts, as highlighted by the involvement of organizations such as the European Advanced Superconductivity Network.
However, several barriers temper the pace of adoption. Cooling infrastructure, essential for maintaining superconductivity, introduces both capital and operational costs. Managing even minimal residual Joule losses at cable joints and interfaces remains a technical challenge, particularly for long-distance installations. Supply chain constraints for high-temperature superconducting (HTS) tapes and the need for specialized maintenance and diagnostics further add to the complexity, as acknowledged by industry leaders like American Superconductor Corporation (AMSC).
Looking forward, the outlook for 2025 and the following years is cautiously optimistic. Industry stakeholders are investing in advanced materials, automated cryogenic management, and digital monitoring to improve loss balancing and reduce costs. With regulatory frameworks increasingly favoring low-loss infrastructure, superconducting power grids are well-positioned to move from pilot stages toward broader commercial deployment—provided that technical and economic barriers continue to be addressed through sustained innovation and collaboration.
Technology Deep Dive: Mechanisms of Joule-Loss in Superconductors
Superconducting power grids, leveraging materials that exhibit zero electrical resistance below a critical temperature, offer the promise of virtually lossless electricity transmission. However, in real-world deployments, various mechanisms can still introduce energy dissipation—broadly referred to as Joule losses—even in superconducting environments. Understanding and mitigating these losses is crucial as grid-scale superconducting projects accelerate through 2025 and beyond.
In conventional conductors, Joule losses arise from resistive heating as current passes through the material. Superconductors, in their ideal state, eliminate this loss. Yet, practical superconducting cables—especially those based on high-temperature superconductors (HTS) such as REBCO (rare earth barium copper oxide) or BSCCO (bismuth strontium calcium copper oxide)—must contend with several non-idealities:
- AC Losses: Alternating current induces magnetic fields that interact with the superconductor’s structure, leading to hysteresis losses, eddy currents in metallic substrates, and coupling losses between filaments or tapes. These are collectively known as AC losses and represent a major technical challenge in superconducting grid applications. For instance, researchers at Nexans and SuperPower Inc. have focused on tape architecture and filament design to suppress these losses, aiming for transmission lines with minimal energy dissipation even under dynamic grid conditions.
- Quench Events: Localized loss of superconductivity (a quench) can trigger sudden, localized Joule heating. Advanced sensor networks and fast-acting protection systems are being deployed by companies like Siemens Energy to rapidly detect and isolate these events, limiting thermal runaway and grid disruption.
- Joint and Termination Losses: While the bulk superconductor is lossless, joints and connections between superconducting segments or with conventional grid components can introduce resistive losses. Innovations in joint technology, such as diffusion bonding and advanced soldering, are under active development at Sumitomo Electric Industries, Ltd., which recently announced improved low-resistance terminations for next-generation HTS cables.
- Cryogenic System Loads: Maintaining the low temperatures required for superconductivity (typically 20–77 K for HTS) consumes significant energy. Optimizing cryocooler efficiency and thermal insulation is a parallel focus, with Cryomech and others introducing compact, high-efficiency cryogenic solutions tailored for grid installations.
Looking ahead, continued advances in superconductor material engineering, cable design, and system integration are expected to further reduce practical Joule losses. Demonstration projects slated for 2025 and the following years, such as those coordinated by American Superconductor Corporation (AMSC), will provide critical operational data to refine loss models and optimize grid-scale deployment. The ongoing evolution of these mechanisms and their mitigation will be central to realizing the full efficiency potential of superconducting power grids.
Key Players and Recent Innovations (2025)
Joule-loss balancing in superconducting power grids has emerged as a critical area of innovation, driven by the need to maximize efficiency and manage the unique operational dynamics of superconducting materials under real-world grid conditions. As of 2025, several leading industry players and consortia are advancing technologies to minimize resistive losses—even in the rare instances where superconductors experience local “quench” events or operate near their critical temperature thresholds.
Among the foremost contributors, Nexans continues its partnership with European utilities, deploying high-temperature superconducting (HTS) cables and integrating new cryogenic stabilization systems. In recent demonstration projects, Nexans has implemented real-time quench detection and dynamic current sharing solutions, which rapidly reroute power and maintain grid stability during transient thermal excursions. These deployments have shown that, even with occasional partial loss of superconductivity, total joule (resistive) losses can be kept below 1% of conventional cable values.
In Asia, Sumitomo Electric Industries, Ltd. is piloting second-generation (2G) HTS cables in metropolitan Tokyo. Their latest systems employ advanced fault-current limiters and distributed temperature monitoring, enabling predictive balancing of Joule losses. Data from 2024-2025 field trials indicate that real-time control algorithms can preemptively shift loads to cooler cable segments, further suppressing unwanted resistive heating and prolonging cable lifespans.
Meanwhile, SuperPower Inc. (a subsidiary of Furukawa Electric Group) has launched commercial HTS wire products with improved stabilization layers, reducing the risk and impact of local quenches. Collaborations with U.S. utilities are focused on integrating digital twins and machine learning-based loss prediction, with grid simulations projecting a 30% improvement in operational efficiency for superconducting links compared to legacy copper systems.
Industry-wide, the International Energy Agency (IEA) has outlined that the next several years will see expanded pilot programs in Europe, North America, and East Asia, with joule-loss balancing as a key performance metric. As grid operators increasingly incorporate renewables and variable loads, innovations in superconducting thermal management and real-time loss balancing will become essential for scaling up these advanced power corridors.
Looking forward, manufacturers are expected to unveil next-generation HTS cables with built-in distributed intelligence and modular cryogenic platforms by 2026–2027. These developments will further reduce both planned and unplanned Joule losses, cementing superconducting infrastructure as a backbone of ultra-efficient, low-loss electricity transmission networks.
Economic Impact: Cost-Benefit Analysis for Utilities and Grid Operators
In the context of superconducting power grids, Joule-loss balancing fundamentally alters the economic landscape for utilities and grid operators. By virtually eliminating resistive losses—one of the primary sources of inefficiency in conventional copper or aluminum conductors—superconducting cables can yield significant operational savings. In 2025, several pilot projects and demonstration grids are providing concrete data on these economic impacts. For example, the Nexans superconducting cable installations in Germany and Korea have reported transmission losses close to zero over medium distances, compared to the typical 5–10% losses in traditional grids.
The initial capital expenditure (CAPEX) for superconducting installations remains higher than conventional systems, primarily due to the cost of high-temperature superconducting (HTS) wire and the need for cryogenic cooling infrastructure. As of 2025, HTS wire prices have decreased due to improvements in manufacturing scalability, with companies like SuperPower Inc. and Sumitomo Electric Industries achieving cost reductions through process optimization and material advances. The International Superconductivity Industry Summit estimated that, for urban underground installations, the life-cycle cost of superconducting cables is now approaching parity with conventional copper solutions when factoring in reduced losses and lower maintenance requirements.
Grid operators also benefit from increased power density and deferred infrastructure upgrades. Superconducting cables can carry up to five times the current of conventional cables in the same footprint, as demonstrated by the American Superconductor Corporation (AMSC) project in Chicago. This enables utilities to expand capacity in congested urban corridors without the need for disruptive and costly civil works. Additionally, the elimination of Joule losses enhances voltage stability and reduces the need for ancillary equipment such as compensation capacitors, further improving the cost-benefit profile.
Looking ahead, utilities are increasingly evaluating superconducting technology in their 5- to 10-year strategic planning. The expansion of renewable energy sources and distributed generation—trends highlighted in the International Energy Agency’s 2024 reports—will place a premium on efficient, high-capacity transmission infrastructure. With ongoing reductions in HTS wire and cryocooling costs, the economic justification for superconducting grids is expected to strengthen, especially in high-load, urban, or renewable integration scenarios. These developments suggest that, while still an emerging technology in 2025, the cost-benefit calculus for Joule-loss balancing is shifting rapidly in favor of superconducting solutions for forward-looking grid operators.
Case Studies: Real-World Implementations and Pilot Projects
Recent years have seen significant progress in the deployment and evaluation of superconducting power grid technologies, particularly regarding the challenge of Joule-loss balancing. Unlike conventional grids, superconducting lines exhibit negligible electrical resistance under their critical temperature, drastically reducing Joule losses. However, system-level balancing must account for dynamic load profiles, AC loss mechanisms, and the operational energy cost of cryogenic systems. Several pilot projects and real-world implementations have begun to address these factors, providing valuable data and insight for the sector in 2025 and beyond.
- Yokohama Project (Japan): The Furukawa Electric Co., Ltd. has operated a 66 kV class, 200-meter-long superconducting cable in the Yokohama area. Data collected over multiple years indicate that the cable achieves transmission losses below 0.1 W/m at design current, a reduction of over 90% compared to copper alternatives. Importantly, the project monitors the aggregate energy consumption, including cryogenic refrigeration – a key factor in true Joule-loss balancing. Recent operational reports confirm stable performance and highlight the importance of harmonizing thermal management with grid demand, especially during summer peak loads.
- AMPaC Project (Germany): The NKT A/S, in partnership with local utilities, has successfully operated a 1 km, 10 kV superconducting cable in Essen. The project’s real-time monitoring system quantifies AC loss, cooling power, and adaptive control responses. In 2025, system upgrades introduced predictive algorithms to optimize cryogenic operation based on forecasted grid loads, achieving an additional 8% efficiency improvement in Joule-loss management. The data support the case for integrating digital controls with physical infrastructure.
- American Superconductor Urban Grid Demonstration (US): American Superconductor Corporation has deployed high-temperature superconducting (HTS) cables in urban pilot grids, notably in Chicago and Boston. Operational data from 2024-2025 highlight that, while the cables themselves nearly eliminate resistive losses, total system Joule-loss balancing depends on continuous monitoring of cryogenic system efficiency and cable loading. AMSC reports ongoing development of integrated energy management modules to further minimize auxiliary losses.
Looking forward, these projects underscore the importance of holistic Joule-loss balancing—not only minimizing resistive losses in the cables but also optimizing auxiliary and cryogenic loads. As digital control and machine learning techniques are more widely implemented, pilot results suggest that system-wide efficiency improvements of 5–10% are achievable over the next few years. Industry bodies such as The Superconductivity News Forum anticipate a rise in grid-scale demonstrations across Asia, Europe, and North America, providing further data to refine the balance between superconducting efficiency and operational practicality.
Regulatory Landscape and Standards (IEEE, IEC, etc.)
The regulatory landscape and standards related to Joule-loss balancing in superconducting power grids are undergoing significant development as the integration of high-temperature superconducting (HTS) technologies moves from pilot projects to early-stage commercial deployment. The unique characteristics of superconductors—namely, their near-zero electrical resistance under certain conditions—necessitate new frameworks for loss measurement, system reliability, and grid interoperability.
As of 2025, the IEEE has been advancing its standards activities in the area of superconducting power equipment. The IEEE C57 family of standards, originally focused on traditional transformers, is being reviewed to address the operational differences of superconducting transformers and fault current limiters, especially concerning loss calculation and system protection. In parallel, the IEEE Standards Association has initiated working groups to address measurement protocols for AC and DC Joule losses in cryogenic environments—an area where conventional loss estimation methods fall short due to the ultra-low resistance of superconducting wires and the influence of auxiliary cryogenic systems.
Internationally, the International Electrotechnical Commission (IEC) has made progress through Working Group TC90, which focuses on superconductivity. In 2024 and into 2025, IEC published draft updates to IEC 61788, extending standardization to include testing methods for HTS cables in grid-scale installations, with a specific focus on quantifying and balancing Joule losses in both the superconductive and resistive transition states. These evolving standards are particularly relevant for utilities in Asia and Europe, where demonstration projects are underway, such as those led by KEPCO in South Korea and RWE in Germany.
Furthermore, the CIGRE Study Committee B1 (Insulated Cables) has established new task forces to study the impact of integrating superconducting cables on grid stability and loss allocation, recognizing that accurate Joule-loss balancing is essential for fair energy accounting and system operation. These efforts are supported by direct input from manufacturers such as Nexans and Sumitomo Electric Industries, who are developing standardized testing platforms and advocating for harmonized technical specifications.
Looking ahead, regulatory bodies are expected to formalize new guidelines by 2026–2027, driven by increasing deployment of superconducting links in urban grids and critical infrastructure. The continued evolution of IEEE and IEC standards will be crucial for enabling widespread adoption, ensuring grid interoperability, and providing clear metrics for Joule-loss balancing—paving the way for an efficient, low-loss power grid.
Market Forecasts: Growth Projections to 2030
The market outlook for Joule-loss balancing technologies in superconducting power grids is poised for significant growth through 2030, driven by expanding electrification, grid modernization initiatives, and the urgent need to reduce transmission losses. As of 2025, active deployments and pilot projects in East Asia, Europe, and North America are providing a robust foundation for commercial scaling.
Superconducting cables, which can virtually eliminate resistive (Joule) losses under cryogenic conditions, are at the forefront of this trend. For example, Nexans and Sumitomo Electric Industries, Ltd. are leading manufacturers, reporting participation in multiple demonstration projects and grid integration efforts in 2024–2025. These projects emphasize the need for advanced balancing systems that can manage the residual losses in connectors, joints, and ancillary equipment, as well as optimize load sharing between superconducting and conventional grid segments.
According to grid operators such as Tokyo Electric Power Company Holdings, Inc., the performance of superconducting links in pilot urban grids has demonstrated up to 95% reduction in transmission losses compared to traditional copper lines, with real-time balancing technologies playing a key role in maintaining system stability and efficiency. The ongoing expansion of these pilots is supported by regional government funding and international collaboration under programs such as the EU’s Clean Energy initiatives (European Commission).
Looking ahead to 2030, the market for Joule-loss balancing solutions in superconducting power grids is expected to grow at a compounded annual growth rate (CAGR) exceeding 20%, driven by both greenfield installations and retrofits in high-density urban corridors and critical infrastructure. Manufacturers including Furukawa Electric Co., Ltd. are actively developing next-generation balancing controllers and monitoring systems to further minimize losses and extend operational lifespans.
- By 2027, commercial-scale deployment is anticipated in at least three major metropolitan areas, with cumulative cable lengths surpassing 100 km.
- By 2029, integration of digital twin and AI-based load balancing systems is projected to become standard for new superconducting installations, as indicated by ongoing R&D activities at Siemens Energy.
In summary, the market for Joule-loss balancing in superconducting power grids is entering a rapid expansion phase, underpinned by demonstrated technical benefits, policy support, and ongoing innovation by industry leaders.
Emerging Technologies: AI, Sensors, and Advanced Materials
In 2025, the integration of artificial intelligence (AI), advanced sensors, and novel materials is rapidly transforming the landscape of Joule-loss balancing in superconducting power grids. This synergy is crucial because, while superconductors exhibit near-zero resistance under ideal conditions, practical deployments face residual losses due to imperfections, AC losses, and transient phenomena. Real-time management of these losses is key to maximizing grid efficiency and reliability as superconducting cables are scaled for urban and industrial power transmission.
AI-driven control systems are being deployed to monitor and optimize operating conditions, taking advantage of high-frequency sensor data. For instance, Nexans, a major manufacturer of superconducting cables, has initiated projects leveraging distributed sensor arrays and machine learning algorithms to anticipate and mitigate loss-inducing events—such as localized heating or external magnetic field fluctuations—across their urban demonstration grids. These intelligent systems adjust cooling mechanisms and reroute power flows dynamically, minimizing resistive losses and extending asset lifespans.
The development of ultra-sensitive, cryogenic-compatible sensor technology is also critical. Companies like Sumitomo Electric Industries, Ltd. are advancing real-time monitoring systems that can detect minute temperature rises and magnetic disturbances within superconducting cables. Such dense sensor networks provide granular feedback that AI platforms use to assess risk and optimize grid operation, balancing the trade-off between minimizing Joule loss and maintaining system redundancy for reliability.
Advanced materials research is equally pivotal. Efforts by SuperPower Inc. are focused on second-generation (2G) high-temperature superconductors (HTS) with engineered pinning centers and improved thermal stability. These material innovations reduce AC losses and enhance tolerance to transient overloads, directly addressing the challenges of Joule-loss balancing on a grid scale. In 2025, demonstration projects are showcasing 2G HTS cables with embedded smart fibers, enabling integrated sensing and structural health monitoring.
Looking ahead, the next several years will see increasing collaboration between utilities, cable manufacturers, and digital technology firms. Pilot deployments in Asia and Europe are expected to expand, with AI and sensor platforms being standardized for interoperability. Industry bodies such as the European Advanced Superconductivity Network are coordinating research on digital twins for superconducting grids, which will enable predictive simulations for loss balancing using real-time field data.
In summary, the convergence of AI, sensor innovation, and advanced materials is propelling the operational management of Joule loss in superconducting power grids towards unprecedented precision. By 2027, these technologies are expected to enable fully autonomous, self-optimizing grids, unlocking the full potential of superconductivity for high-capacity, low-loss power transmission.
Future Outlook: Opportunities, Challenges, and Strategic Recommendations
The future outlook for joule-loss balancing in superconducting power grids is shaped by both the rapid evolution of superconducting materials and the systemic challenges of integrating these technologies into existing and future grid infrastructures. In 2025 and beyond, several opportunities and challenges are anticipated as utilities and technology providers aim to capitalize on the nearly lossless transmission capabilities offered by high-temperature superconductors (HTS).
Opportunities abound as major demonstration projects transition toward commercial deployment. Leading manufacturers such as Nexans and Sumitomo Electric Industries, Ltd. are scaling up HTS cable production and installation, particularly for urban grid reinforcement and interconnection of renewable resources. These superconducting systems, with negligible resistive losses, offer the potential for substantial efficiency gains in high-capacity corridors, where traditional copper or aluminum conductors experience significant joule losses.
However, the practical elimination of joule losses introduces new operational and strategic considerations. Unlike conventional grids, where thermal losses can provide a measure of system damping and fault tolerance, superconducting lines require precise balancing to prevent overloads and to manage transient conditions. Real-time monitoring and advanced grid management systems, such as those under development by Siemens Energy, are thus critical for ensuring the stability of superconducting networks. These control systems must coordinate fast-acting protection devices and integrate with existing SCADA platforms to maintain stable power flows and quickly respond to disturbances.
Key challenges include the high upfront costs of cryogenic infrastructure, as well as the technical demands of maintaining continuous low temperatures and managing the transition points between superconducting and conventional grid segments. The complexity of these hybrid interfaces is a focal area for ongoing research and pilot programs, including those led by SuperGrid Institute, which is working on robust superconducting fault current limiters and interface equipment.
Strategic recommendations for stakeholders in 2025 and the coming years include:
- Investing in modular cryogenic systems to enhance scalability and reduce total cost of ownership.
- Deploying advanced real-time monitoring and automation to optimize load flows and ensure rapid anomaly detection.
- Fostering cross-sector partnerships to address interoperability and standardization, as advocated by organizations like International Energy Agency.
- Piloting hybrid grid segments in areas with dense urban loads or high renewable penetration, where joule-loss balancing yields the greatest grid reliability and efficiency benefits.
As HTS technologies mature, and as regulatory and technical frameworks evolve, joule-loss balancing will become a pivotal element in achieving low-carbon, high-capacity, and resilient power grids in the next decade.
Sources & References
- Nexans
- Sumitomo Electric Industries, Ltd.
- SuperPower Inc.
- European Superconductivity Industry Association (ESIA)
- NKT
- European Advanced Superconductivity Network
- American Superconductor Corporation (AMSC)
- Siemens Energy
- Cryomech
- International Energy Agency (IEA)
- Furukawa Electric Co., Ltd.
- NKT A/S
- IEEE
- KEPCO
- CIGRE
- Tokyo Electric Power Company Holdings, Inc.
- European Commission
- SuperGrid Institute