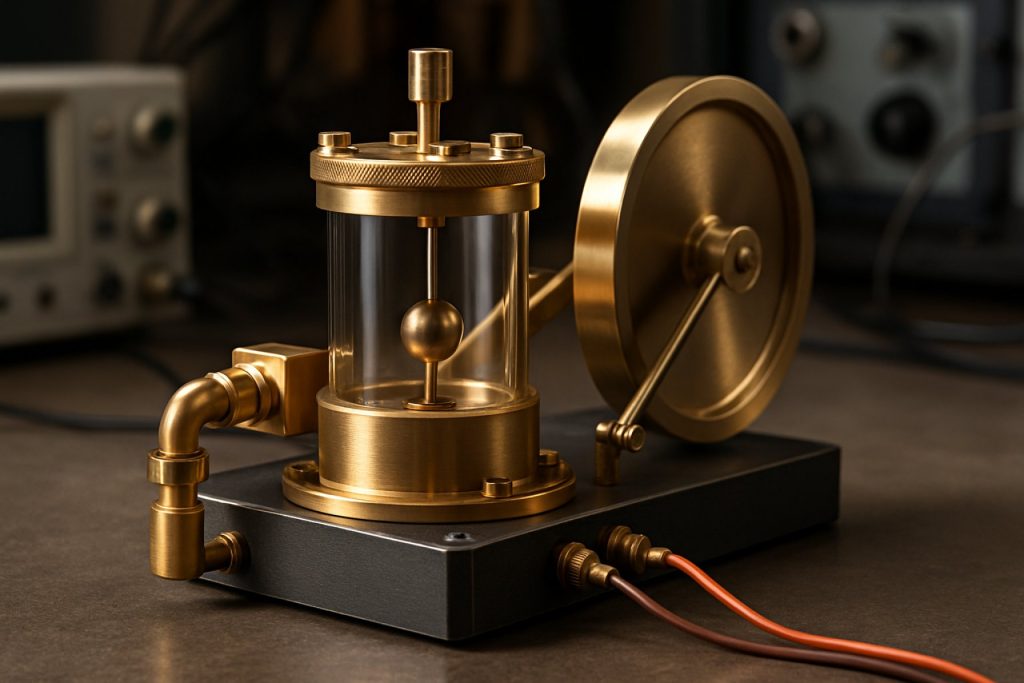
Unlocking the Secrets of the Szilard Engine: How a Single-Particle Device Challenges the Foundations of Physics. Explore Its Impact on Information Theory, Quantum Technology, and the Future of Energy. (2025)
- Introduction: The Origin and Concept of the Szilard Engine
- Szilard Engine and Maxwell’s Demon: Bridging Physics and Information
- Thermodynamics Revisited: Entropy, Information, and the Second Law
- Experimental Realizations: From Theory to Laboratory Demonstrations
- Quantum Szilard Engines: Extending the Model to the Quantum Realm
- Technological Implications: Nanoscale Machines and Information Processing
- Szilard Engine in Modern Research: Key Studies and Breakthroughs
- Public and Academic Interest: Growth Trends and Forecasts
- Challenges and Controversies: Debates in Physics and Engineering
- Future Outlook: Potential Applications and the Road Ahead
- Sources & References
Introduction: The Origin and Concept of the Szilard Engine
The Szilard engine, first conceptualized in 1929 by Hungarian-American physicist Leo Szilard, stands as a foundational thought experiment in the intersection of thermodynamics, information theory, and quantum mechanics. Szilard’s original proposal, published in the journal Zeitschrift für Physik, was designed to probe the paradoxes of Maxwell’s demon—a hypothetical being that seemingly violates the second law of thermodynamics by sorting fast and slow molecules to decrease entropy without expending energy. Szilard’s engine distilled this paradox into its simplest form: a single-molecule gas in a box, with a movable partition and a “demon” that observes the molecule’s position and uses this information to extract work from thermal fluctuations.
The core concept of the Szilard engine is elegantly simple yet profound. By inserting a partition into a box containing a single molecule, and then determining on which side the molecule resides, the “demon” can allow the molecule to push the partition, performing work as it expands isothermally. This process appears to convert information (the knowledge of the molecule’s position) directly into usable energy, challenging the classical understanding of entropy and the inviolability of the second law of thermodynamics. Szilard’s analysis, however, revealed that the act of measurement and the subsequent erasure of information by the demon incurs a thermodynamic cost, thus preserving the second law when information processing is properly accounted for.
The Szilard engine’s significance extends far beyond its original context. It laid the groundwork for the modern field of information thermodynamics, influencing the development of concepts such as Landauer’s principle, which quantifies the minimum energy required to erase a bit of information. The engine also serves as a bridge between classical and quantum physics, inspiring experimental realizations in both regimes and prompting ongoing debates about the physical nature of information. Today, the Szilard engine is frequently cited in discussions of quantum information, nanotechnology, and the fundamental limits of computation.
Leo Szilard himself was a prominent figure in 20th-century physics, contributing to nuclear chain reaction theory and advocating for the responsible use of scientific discoveries. His engine remains a touchstone in the study of the deep connections between physics and information, and continues to inspire research at leading institutions such as the American Physical Society and the American Institute of Physics.
Szilard Engine and Maxwell’s Demon: Bridging Physics and Information
The Szilard engine, proposed by physicist Leo Szilard in 1929, is a conceptual device that elegantly bridges the domains of thermodynamics and information theory. It was designed as a simplified, one-molecule analog of James Clerk Maxwell’s famous “demon” thought experiment, which challenged the second law of thermodynamics by suggesting that information could be used to decrease entropy. Szilard’s model consists of a single gas molecule in a box, a movable partition, and a hypothetical “demon” capable of observing the molecule’s position and manipulating the partition accordingly.
The operation of the Szilard engine proceeds in several steps. First, the demon inserts a partition into the box, dividing it into two equal volumes. By measuring which side the molecule occupies, the demon gains one bit of information. The demon then allows the molecule to push the partition, extracting work from the system as the molecule expands isothermally against the partition. This process appears to convert information about the molecule’s position directly into usable work, seemingly violating the second law of thermodynamics.
Szilard’s critical insight was to recognize that the act of measurement and the subsequent erasure of information are not thermodynamically free. In particular, the erasure of the demon’s memory—resetting it to a standard state—incurs a minimum energy cost, as formalized later by Rolf Landauer in the 1960s. This cost, known as Landauer’s principle, states that erasing one bit of information dissipates at least ( k_B T ln 2 ) of energy as heat, where ( k_B ) is Boltzmann’s constant and ( T ) is the temperature of the heat bath. Thus, when the full thermodynamic cycle is considered, including information processing, the second law remains intact.
The Szilard engine has become a foundational model in the field of information thermodynamics, influencing both theoretical and experimental research. It has inspired studies in the physics of computation, the thermodynamics of small systems, and the energetic costs of information processing. Modern experiments with colloidal particles and optical traps have realized Szilard-like engines at the microscale, confirming the theoretical predictions and deepening our understanding of the interplay between information and energy. The engine’s legacy is evident in the ongoing work of organizations such as the American Physical Society and the Institute of Physics, which continue to support research at the intersection of physics and information science.
Thermodynamics Revisited: Entropy, Information, and the Second Law
The Szilard engine, first conceptualized by physicist Leo Szilard in 1929, stands as a pivotal thought experiment at the intersection of thermodynamics and information theory. Szilard’s model was designed to probe the foundations of the second law of thermodynamics, particularly in the context of Maxwell’s demon—a hypothetical being capable of violating the law by sorting particles to decrease entropy without expending energy. The Szilard engine simplifies this scenario to a single-particle gas in a box, partitioned by a movable wall, and demonstrates how information acquisition and processing are fundamentally linked to thermodynamic entropy.
In the Szilard engine, a single molecule is trapped in a cylinder connected to a heat reservoir. A partition is inserted, and the position of the molecule (left or right) is measured. Based on this information, the partition is allowed to move, extracting work from the system as the molecule pushes against it. The key insight is that the act of measurement—gaining information about the molecule’s position—enables the extraction of kT ln(2) amount of work (where k is Boltzmann’s constant and T is temperature) from the heat bath. This process appears to challenge the second law, which states that entropy in a closed system cannot decrease.
However, Szilard’s analysis, later refined by Rolf Landauer and Charles Bennett, revealed that the second law remains intact when the full thermodynamic cycle is considered. The crucial step is the erasure of information: resetting the demon’s memory to its original state incurs a minimum entropy cost, as articulated by Landauer’s principle. This principle asserts that erasing one bit of information increases the entropy of the environment by at least k ln(2), thus preserving the second law. The Szilard engine thus illustrates that information is a physical quantity, and its manipulation has unavoidable thermodynamic consequences.
- The American Physical Society has published numerous studies and reviews on the Szilard engine, highlighting its role in the development of modern statistical mechanics and information thermodynamics.
- The National Institute of Standards and Technology has contributed to experimental realizations of information engines, validating the theoretical predictions of Szilard and Landauer.
- The American Physical Society and NIST both emphasize the Szilard engine’s importance in understanding the physical nature of information and its implications for the second law of thermodynamics.
In summary, the Szilard engine remains a foundational model for exploring the deep connections between entropy, information, and the second law. Its legacy endures in contemporary research on quantum information, computation, and the thermodynamics of small systems.
Experimental Realizations: From Theory to Laboratory Demonstrations
The Szilard engine, first conceptualized by physicist Leo Szilard in 1929, has long served as a theoretical touchstone in discussions of the relationship between information and thermodynamics. The original thought experiment posited a single-molecule gas in a box, with a partition and a “demon” capable of extracting work by making measurements and manipulating the system. For decades, the Szilard engine remained a theoretical construct, but advances in experimental physics and nanotechnology have enabled laboratory demonstrations that bring Szilard’s ideas into the realm of empirical science.
The first experimental realizations of Szilard-like engines emerged in the early 21st century, leveraging optical tweezers and colloidal particles to mimic the single-molecule scenario. In these setups, a microscopic bead suspended in a fluid is trapped and manipulated using highly focused laser beams. By monitoring the bead’s position and applying feedback based on real-time measurements, researchers have demonstrated the conversion of information into work, in line with Szilard’s predictions. These experiments have confirmed that the act of measurement and feedback can indeed extract work from a thermal reservoir, but only when the information gained is properly utilized, thus upholding the second law of thermodynamics when the cost of information processing is included.
A landmark experiment was conducted by a team at the University of Tokyo, who used a single colloidal particle in a time-dependent optical trap to realize a Szilard engine. Their results, published in 2010, provided quantitative verification of the theoretical predictions, including the relationship between information and extracted work. Subsequent experiments have refined these techniques, employing more sophisticated feedback protocols and exploring the limits of measurement precision and control. These laboratory realizations have not only validated the theoretical framework but have also deepened our understanding of the thermodynamic cost of information processing, a topic of central importance in the field of information thermodynamics.
Beyond colloidal systems, researchers have explored implementations using single-electron devices, quantum dots, and superconducting circuits. These platforms allow for the investigation of Szilard engine principles at the quantum scale, where quantum measurement and coherence introduce new subtleties. For example, experiments with single-electron boxes have demonstrated the extraction of work from information in solid-state systems, opening avenues for the integration of information engines into future nanoscale technologies.
The experimental realization of the Szilard engine has thus transitioned from a theoretical curiosity to a vibrant area of research, with implications for the foundations of thermodynamics, the physics of computation, and the design of energy-efficient information processing devices. Leading research institutions and organizations such as the RIKEN research institute in Japan and the Max Planck Society in Germany continue to advance this field, exploring both classical and quantum regimes of information-driven engines.
Quantum Szilard Engines: Extending the Model to the Quantum Realm
The Szilard engine, originally conceived by Leo Szilard in 1929, is a thought experiment that explores the relationship between information and thermodynamics. In its classical form, the engine consists of a single-molecule gas in a box, with a partition inserted to extract work based on knowledge of the molecule’s position. This model has been pivotal in discussions about Maxwell’s demon and the thermodynamic cost of information processing. In recent years, the concept has been extended into the quantum domain, giving rise to the quantum Szilard engine—a system that leverages quantum properties such as superposition, entanglement, and measurement-induced state changes.
Quantum Szilard engines differ fundamentally from their classical counterparts due to the unique features of quantum mechanics. In the quantum version, the working substance (often a single atom or particle) can exist in a superposition of states, and the act of measurement itself can alter the system’s state. This introduces new considerations regarding the extraction of work and the role of information. For instance, quantum measurements can be invasive, collapsing the wavefunction and potentially reducing the extractable work compared to the classical case. However, quantum correlations and entanglement can also enable new modes of operation, sometimes allowing for work extraction that would be impossible classically.
Theoretical studies have shown that the maximum work extractable from a quantum Szilard engine is governed by the von Neumann entropy, the quantum analogue of classical entropy. This links the engine’s performance directly to the information content of the quantum state. Furthermore, the quantum Szilard engine has become a testbed for exploring the thermodynamics of quantum information, including the cost of quantum measurements and the role of feedback control. These investigations are central to the emerging field of quantum thermodynamics, which seeks to generalize the laws of thermodynamics to quantum systems.
Experimental realizations of quantum Szilard engines are challenging but have become increasingly feasible with advances in quantum technologies. Systems such as trapped ions, superconducting qubits, and ultracold atoms are being used to simulate and test the principles underlying quantum engines. These platforms are developed and maintained by leading research institutions and organizations, including National Institute of Standards and Technology and Max Planck Society, which are at the forefront of quantum information science. The insights gained from quantum Szilard engines are expected to inform the design of future quantum devices, including quantum computers and nanoscale engines, where the interplay between information and energy is of paramount importance.
Technological Implications: Nanoscale Machines and Information Processing
The Szilard engine, first conceptualized by physicist Leo Szilard in 1929, remains a foundational thought experiment in the intersection of thermodynamics, information theory, and the physics of computation. The engine demonstrates how information about a system’s microscopic state can, in principle, be converted into useful work, challenging the classical understanding of the second law of thermodynamics. In recent years, advances in nanotechnology and quantum information science have transformed the Szilard engine from a theoretical construct into a practical framework for exploring the limits of energy conversion and information processing at the nanoscale.
At the heart of the Szilard engine is the idea that measurement and information acquisition can have thermodynamic consequences. This insight has profound implications for the design of nanoscale machines, where thermal fluctuations and quantum effects become significant. Modern research has realized physical analogs of the Szilard engine using single-electron boxes, optical traps, and quantum dots, allowing experimentalists to probe the energetic cost of measurement and feedback at the level of individual particles. These experiments have confirmed that the act of acquiring and erasing information is fundamentally linked to entropy production, as formalized by Landauer’s principle, which states that erasing one bit of information requires a minimum energy cost of kT ln 2, where k is Boltzmann’s constant and T is temperature.
The technological implications of these findings are far-reaching. In the realm of nanoscale machines, the Szilard engine provides a blueprint for designing devices that harness information to perform work with maximal efficiency. Such principles are being explored in the development of molecular motors, artificial nanorobots, and energy-harvesting systems that operate close to the thermodynamic limits. For example, researchers are investigating how feedback-controlled molecular systems can rectify thermal noise to drive directed motion or chemical reactions, potentially revolutionizing fields such as targeted drug delivery and synthetic biology.
In information processing, the Szilard engine underscores the physical nature of computation. As devices shrink to the atomic scale, the energetic cost of logical operations and data storage becomes a critical design constraint. Theoretical and experimental studies inspired by the Szilard engine are guiding the development of ultra-low-power computing architectures, including reversible and quantum computing, where minimizing heat dissipation is essential for scalability and performance. Organizations such as the Institute of Electrical and Electronics Engineers (IEEE) and the American Physical Society (APS) are actively supporting research at this intersection of physics, information, and technology.
As we move into 2025, the Szilard engine continues to inspire new paradigms in nanoscale engineering and information science, highlighting the deep connections between knowledge, control, and the fundamental limits of technology.
Szilard Engine in Modern Research: Key Studies and Breakthroughs
The Szilard engine, first conceptualized by physicist Leo Szilard in 1929, has become a cornerstone in the study of the relationship between information and thermodynamics. In recent years, modern research has revitalized interest in the Szilard engine, particularly as it relates to the physical limits of computation, the role of information in entropy, and the foundations of quantum thermodynamics. The engine’s theoretical framework—where a single-molecule gas in a box is manipulated using information about its position—has inspired a new generation of experimental and theoretical studies.
One of the most significant breakthroughs in the 21st century has been the experimental realization of Szilard-type engines at the microscopic scale. Researchers have constructed single-particle systems using optical traps and feedback mechanisms to mimic the original Szilard engine, directly demonstrating the conversion of information into work. These experiments have validated the predictions of information thermodynamics, showing that the acquisition and use of information can indeed reduce entropy and extract work, in accordance with Landauer’s principle. Notably, studies published by leading physics research institutions have confirmed that the minimum energy cost of erasing information is fundamentally linked to the second law of thermodynamics.
In the quantum domain, the Szilard engine has become a testbed for exploring the interplay between quantum measurement, feedback, and thermodynamic laws. Quantum versions of the engine have been proposed and, in some cases, realized using superconducting qubits and trapped ions. These systems allow researchers to probe the effects of quantum coherence and entanglement on the efficiency and operation of information engines. Theoretical work by organizations such as the American Physical Society and experimental collaborations at major research universities have advanced our understanding of how quantum information can be harnessed to perform work, and how the act of measurement itself influences thermodynamic outcomes.
Recent reviews and meta-analyses by the American Physical Society and the Institute of Physics highlight the Szilard engine’s role in bridging classical and quantum thermodynamics, and its implications for the development of future nanoscale machines and quantum computers. As of 2025, ongoing research continues to push the boundaries of what is possible, with new experimental platforms and theoretical models deepening our understanding of the fundamental links between information, entropy, and energy.
Public and Academic Interest: Growth Trends and Forecasts
The Szilard engine, a conceptual device introduced by physicist Leo Szilard in 1929, has experienced a resurgence of public and academic interest in recent years, particularly as the intersection of thermodynamics, information theory, and quantum mechanics becomes increasingly relevant to emerging technologies. The Szilard engine, which demonstrates the conversion of information into work, has become a focal point for research into the fundamental limits of computation and the physical nature of information.
Academic interest in the Szilard engine has grown steadily, as evidenced by the increasing number of peer-reviewed publications and conference presentations dedicated to the topic. This growth is driven by the engine’s role as a model system for exploring Maxwell’s demon paradox and the thermodynamic cost of information processing. Leading research institutions and universities worldwide have established dedicated research groups and interdisciplinary collaborations to investigate the implications of the Szilard engine for quantum information science, nanotechnology, and the development of energy-efficient computing systems.
Forecasts for 2025 suggest that research activity related to the Szilard engine will continue to expand, propelled by advances in experimental techniques that allow for the realization of Szilard-type engines at the nanoscale. Theoretical developments, particularly in the context of quantum thermodynamics, are expected to further deepen our understanding of the relationship between information and energy. Funding agencies and scientific organizations, such as the National Science Foundation and the European Organization for Nuclear Research (CERN), have recognized the significance of this research area, supporting projects that explore the practical and foundational aspects of information engines.
Public interest in the Szilard engine is also on the rise, fueled by popular science outreach and the growing awareness of the importance of energy efficiency in computation. Educational platforms and science museums increasingly feature the Szilard engine in exhibits and lectures, highlighting its relevance to both historical and contemporary scientific challenges. As quantum computing and artificial intelligence become more prominent in public discourse, the Szilard engine serves as an accessible entry point for discussions about the physical limits of computation and the role of information in the universe.
In summary, the Szilard engine is poised to remain a central topic in both academic research and public science education through 2025 and beyond, with growth trends reflecting its foundational importance to multiple scientific disciplines and its potential impact on future technologies.
Challenges and Controversies: Debates in Physics and Engineering
The Szilard engine, first conceptualized by physicist Leo Szilard in 1929, remains a focal point of debate in both physics and engineering, particularly regarding the fundamental limits of thermodynamics and the role of information in physical systems. The engine is a thought experiment that demonstrates how information about a single molecule’s position could, in principle, be used to extract work from a heat bath, seemingly challenging the second law of thermodynamics. This paradox has sparked extensive theoretical and experimental scrutiny, especially as advances in nanotechnology and quantum information science bring such concepts closer to practical realization.
One of the central challenges is reconciling the Szilard engine with the second law of thermodynamics. The engine appears to allow for the extraction of work without a corresponding increase in entropy, which would violate the law. However, subsequent analyses, particularly those incorporating the role of measurement and information erasure, have shown that the total entropy of the system, including the observer or “demon,” does not decrease. The process of acquiring and erasing information is now understood to have thermodynamic costs, as formalized by Landauer’s principle, which states that erasing one bit of information increases the entropy of the environment by at least k ln 2, where k is Boltzmann’s constant. This principle has been experimentally verified in recent years, reinforcing the compatibility of the Szilard engine with established thermodynamic laws (American Physical Society).
Another controversy involves the practical implementation of Szilard-like engines at the nanoscale. While the original engine was a thought experiment, modern advances in micro- and nano-fabrication have enabled the construction of physical systems that mimic its operation. These experiments, often involving single-electron boxes or optical traps, have provided valuable insights but also highlighted engineering challenges such as thermal fluctuations, measurement precision, and the energetic cost of feedback control. The National Institute of Standards and Technology (NIST) and other leading research institutions have conducted experiments demonstrating the conversion of information to work, but scaling these systems for practical energy harvesting remains a significant hurdle.
Debates also persist regarding the interpretation of information in physical systems. Some physicists argue that information is a purely abstract concept, while others contend that it has tangible physical consequences, as exemplified by the Szilard engine. This ongoing discourse influences research in quantum thermodynamics, where the interplay between information, measurement, and energy is even more nuanced due to quantum coherence and entanglement effects.
In summary, the Szilard engine continues to challenge and refine our understanding of the relationship between information and thermodynamics. While theoretical and experimental progress has resolved some controversies, particularly regarding the second law, ongoing research in both physics and engineering is required to address the practical and conceptual challenges that remain.
Future Outlook: Potential Applications and the Road Ahead
The Szilard engine, first conceptualized by physicist Leo Szilard in 1929, remains a cornerstone in the ongoing exploration of the relationship between information and thermodynamics. As we look toward 2025 and beyond, the future outlook for the Szilard engine is shaped by advances in quantum information science, nanotechnology, and the deepening understanding of the physical limits of computation. The Szilard engine’s theoretical framework—where a single molecule’s position is measured and manipulated to extract work—has inspired a new generation of research into the fundamental connections between information, entropy, and energy.
One of the most promising potential applications lies in the development of ultra-efficient nanoscale engines and information-driven devices. As researchers continue to miniaturize mechanical systems, the principles underlying the Szilard engine could inform the design of molecular machines that operate at or near the thermodynamic limits of efficiency. Such devices may find use in fields ranging from targeted drug delivery to energy harvesting at the nanoscale. The National Institute of Standards and Technology (NIST), for example, is actively involved in research on the thermodynamics of small systems, exploring how information can be harnessed to control energy flows at the molecular level.
In quantum information science, the Szilard engine serves as a model for understanding the energetic costs of measurement and feedback in quantum systems. As quantum computing and quantum communication technologies advance, the insights derived from Szilard engine experiments are expected to play a crucial role in optimizing the energy efficiency of quantum devices. Organizations such as the Centre for Quantum Technologies are at the forefront of investigating the interplay between information theory and thermodynamics, with the Szilard engine frequently cited as a foundational example.
Looking ahead, the road for practical Szilard engine applications is not without challenges. Realizing functional engines at the molecular or quantum scale requires overcoming significant technical hurdles, including precise measurement, control, and error correction in noisy environments. However, ongoing interdisciplinary collaborations between physicists, engineers, and information theorists are steadily advancing the field. The continued support from major scientific bodies, such as the American Physical Society, ensures that research into the Szilard engine and its implications for the future of energy, computation, and information processing will remain a vibrant and evolving area of inquiry.
Sources & References
- National Institute of Standards and Technology
- RIKEN
- Max Planck Society
- Institute of Electrical and Electronics Engineers
- National Science Foundation
- European Organization for Nuclear Research (CERN)
- Centre for Quantum Technologies
https://youtube.com/watch?v=4DBZcA677Mw