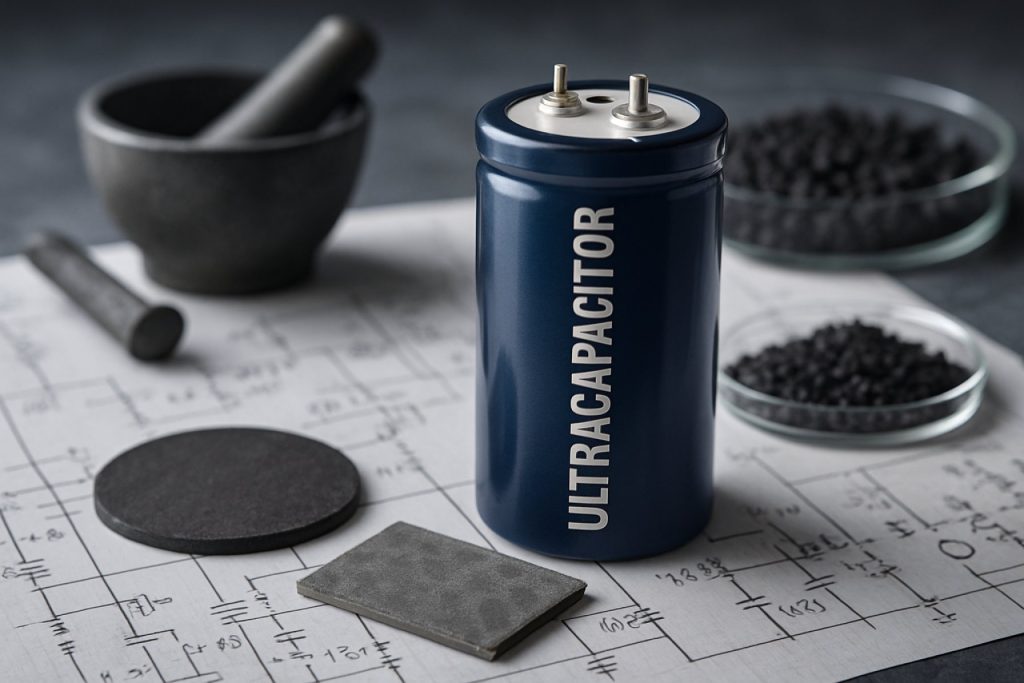
Ultracapacitor Materials Engineering in 2025: How Next-Gen Materials Are Accelerating Energy Storage Innovation and Driving a Projected 40% Market Surge by 2030. Explore the Technologies, Players, and Trends Shaping the Future of High-Performance Capacitors.
- Executive Summary: Market Outlook and Key Drivers (2025–2030)
- Ultracapacitor Fundamentals: Materials and Performance Metrics
- Emerging Materials: Graphene, Carbon Nanotubes, and Beyond
- Electrolyte Innovations and Their Impact on Energy Density
- Manufacturing Advances: Scalability and Cost Reduction
- Key Industry Players and Strategic Partnerships
- Application Trends: Automotive, Grid, and Industrial Sectors
- Regulatory Landscape and Industry Standards (ieee.org, sae.org)
- Market Forecast: Growth Projections and Regional Analysis (2025–2030)
- Future Outlook: R&D Pipelines and Disruptive Technologies
- Sources & References
Executive Summary: Market Outlook and Key Drivers (2025–2030)
The ultracapacitor materials engineering sector is poised for significant growth and transformation between 2025 and 2030, driven by rapid advancements in energy storage technologies and the increasing demand for high-performance, sustainable solutions across automotive, grid, and industrial applications. The market outlook is shaped by the convergence of several key drivers: the electrification of transportation, the proliferation of renewable energy systems, and the global push for decarbonization and energy efficiency.
A central trend is the ongoing innovation in electrode materials, particularly the development and commercialization of advanced carbon-based materials such as graphene, carbon nanotubes, and activated carbons. These materials offer superior surface area, conductivity, and cycle life, directly impacting the energy and power density of ultracapacitors. Leading manufacturers such as Maxwell Technologies (a subsidiary of Tesla, Inc.), Skeleton Technologies, and Eaton Corporation are investing heavily in R&D to optimize material properties and scale up production. For instance, Skeleton Technologies is leveraging patented curved graphene to achieve record-breaking power densities, targeting automotive and grid stabilization markets.
Another key driver is the integration of ultracapacitors with lithium-ion batteries in hybrid energy storage systems, which is accelerating the adoption of advanced materials that can withstand high charge/discharge rates and extreme operating conditions. This trend is particularly evident in electric vehicles (EVs), where ultracapacitors are used for regenerative braking and peak power support. Companies like Maxwell Technologies and Eaton Corporation are actively collaborating with automotive OEMs to develop next-generation modules that combine high energy and power densities.
Supply chain resilience and sustainability are also shaping the market outlook. The industry is increasingly focused on sourcing raw materials responsibly and developing environmentally friendly manufacturing processes. Efforts to reduce reliance on rare or hazardous materials are expected to intensify, with companies exploring bio-derived carbons and water-based electrolytes.
Looking ahead to 2030, the ultracapacitor materials engineering market is expected to benefit from continued policy support for clean energy and electrification, as well as from the maturation of manufacturing technologies that enable cost-effective, high-volume production. The competitive landscape will likely see further consolidation, with established players and innovative startups vying for leadership in materials performance and application-specific solutions. As a result, ultracapacitors are set to play an increasingly critical role in the global energy transition.
Ultracapacitor Fundamentals: Materials and Performance Metrics
Ultracapacitor materials engineering is at the forefront of energy storage innovation in 2025, driven by the demand for higher energy density, longer cycle life, and improved safety. The core components of ultracapacitors—electrodes, electrolytes, and separators—are being reimagined through advanced materials science to meet the evolving requirements of automotive, grid, and industrial applications.
Electrode materials remain the primary focus for performance enhancement. Traditionally, activated carbon has dominated due to its high surface area and cost-effectiveness. However, in 2025, there is a marked shift toward engineered carbons, such as graphene and carbon nanotubes, which offer superior conductivity and tailored pore structures. Companies like Skeleton Technologies are commercializing ultracapacitors based on patented curved graphene, reporting significant improvements in power density and charge/discharge rates. Similarly, Maxwell Technologies (now a part of Tesla) continues to refine carbon-based electrodes for automotive and grid storage solutions.
Beyond carbon, research and early commercialization efforts are exploring transition metal oxides and conducting polymers as pseudocapacitive materials. These materials can store more energy through fast surface redox reactions, potentially bridging the gap between batteries and traditional ultracapacitors. However, challenges remain in terms of cycle stability and scalability, with most commercial products still relying on carbon-based electrodes.
Electrolyte innovation is another critical area. Aqueous electrolytes offer high ionic conductivity and safety but are limited by voltage windows, while organic electrolytes enable higher voltages at the expense of flammability and cost. In 2025, hybrid electrolytes and ionic liquids are gaining traction, aiming to combine safety, voltage, and temperature stability. Companies such as CAP-XX are actively developing and integrating advanced electrolytes to push the operational boundaries of their ultracapacitor modules.
Separator materials, though less publicized, are also evolving. The use of ultra-thin, highly porous polymer membranes is improving ion transport and device reliability. Manufacturers are increasingly collaborating with specialty polymer suppliers to tailor separator properties for specific applications.
Looking ahead, the next few years are expected to see further integration of nanostructured materials and hybrid architectures, with a focus on sustainability and recyclability. The industry outlook is optimistic, with ongoing investments in R&D and manufacturing scale-up by leading players such as Skeleton Technologies, Maxwell Technologies, and CAP-XX, all aiming to deliver ultracapacitors with higher energy densities, longer lifespans, and broader application potential.
Emerging Materials: Graphene, Carbon Nanotubes, and Beyond
The field of ultracapacitor materials engineering is undergoing rapid transformation in 2025, driven by the integration of advanced carbon-based materials such as graphene and carbon nanotubes (CNTs). These materials are at the forefront of efforts to enhance energy density, power delivery, and cycle life, addressing the traditional limitations of ultracapacitors compared to batteries.
Graphene, a single layer of carbon atoms arranged in a hexagonal lattice, is prized for its exceptional electrical conductivity, mechanical strength, and high surface area. In 2025, several manufacturers are scaling up the use of graphene in commercial ultracapacitor electrodes. For instance, Skeleton Technologies has developed proprietary “curved graphene” materials, which they claim significantly increase capacitance and reduce internal resistance, enabling higher power and energy densities. Their ultracapacitors are being deployed in transportation and grid applications, with ongoing R&D focused on further improving electrode formulations.
Carbon nanotubes, with their tubular nanostructure, offer high conductivity and large surface area, making them ideal for double-layer capacitance. Companies such as NAWA Technologies are commercializing vertically aligned CNT electrodes, which reportedly deliver up to ten times the power and energy density of conventional activated carbon-based ultracapacitors. NAWA’s “Ultra Fast Carbon Battery” leverages this architecture, and pilot production lines are being established in Europe to meet growing demand from the automotive and industrial sectors.
Beyond graphene and CNTs, hybrid materials and composites are gaining traction. The integration of pseudocapacitive materials—such as transition metal oxides or conducting polymers—with carbon nanostructures is a key research direction. This approach aims to combine the high power of carbon materials with the higher energy storage of redox-active compounds. Companies like Maxwell Technologies (now a subsidiary of Tesla) are exploring such hybrid electrodes, targeting applications that require both rapid charge/discharge and increased energy density.
Looking ahead, the next few years are expected to see further breakthroughs in scalable synthesis, cost reduction, and integration of these advanced materials into mass-market ultracapacitor products. Industry collaborations with research institutes are accelerating the translation of laboratory advances into manufacturable solutions. As the electrification of transport and renewable energy storage accelerates, the demand for high-performance ultracapacitors is set to grow, with materials engineering at the core of this evolution.
Electrolyte Innovations and Their Impact on Energy Density
Electrolyte innovation is a central driver in advancing ultracapacitor materials engineering, with direct implications for energy density, safety, and operational temperature range. As of 2025, the ultracapacitor industry is witnessing a shift from traditional aqueous and organic electrolytes toward advanced formulations, including ionic liquids, solid-state electrolytes, and hybrid systems. These developments are crucial for bridging the energy density gap between ultracapacitors and batteries, while maintaining the hallmark high power density and cycle life of capacitive storage.
Leading manufacturers such as Maxwell Technologies (a subsidiary of Tesla) and Skeleton Technologies are actively developing and commercializing new electrolyte chemistries. Skeleton Technologies has focused on low-resistance organic electrolytes paired with their patented curved graphene materials, enabling higher voltage operation and improved energy density. Their latest ultracapacitor modules, released in 2024, utilize these innovations to achieve energy densities exceeding 20 Wh/kg, a significant leap from the 5–10 Wh/kg typical of earlier generations.
Ionic liquid electrolytes are gaining traction due to their wide electrochemical stability window (up to 3.5–4 V), non-flammability, and thermal stability. Companies such as Eaton and Skeleton Technologies are exploring these materials for next-generation modules, targeting automotive and grid applications where safety and longevity are paramount. However, challenges remain in terms of ionic conductivity and cost, prompting ongoing research into tailored ionic liquid blends and hybrid electrolytes that combine the best properties of organic solvents and ionic liquids.
Solid-state electrolytes represent another frontier, with research efforts intensifying in 2025. These materials promise to eliminate leakage and flammability risks, potentially enabling ultracapacitors to operate at even higher voltages and temperatures. While commercial deployment is still in its early stages, companies like Maxwell Technologies are reportedly investing in pilot-scale production and collaborative R&D with materials suppliers.
Looking ahead, the next few years are expected to see incremental but impactful improvements in electrolyte formulations. The focus will be on increasing voltage stability, reducing internal resistance, and ensuring compatibility with advanced electrode materials such as graphene and carbon nanotubes. As these innovations mature, ultracapacitors are poised to capture a larger share of the energy storage market, particularly in applications demanding rapid charge/discharge cycles, high reliability, and extended operational lifetimes.
Manufacturing Advances: Scalability and Cost Reduction
The ultracapacitor industry in 2025 is witnessing significant advances in materials engineering, directly impacting manufacturing scalability and cost reduction. The sector’s focus has shifted from laboratory-scale innovation to industrial-scale production, with leading manufacturers and materials suppliers investing in new processes and supply chain integration to meet growing demand for high-performance energy storage.
A central trend is the adoption of advanced carbon materials, such as activated carbon derived from sustainable sources, graphene, and carbon nanotubes. These materials offer high surface area and conductivity, which are critical for increasing energy and power density while maintaining long cycle life. Companies like Maxwell Technologies (now part of Tesla) and Skeleton Technologies are scaling up production of ultracapacitors using proprietary carbon-based electrodes. Skeleton Technologies in particular has commercialized “curved graphene” materials, claiming significant improvements in energy density and cost per kilowatt-hour, and is expanding its manufacturing capacity in Europe to meet automotive and grid storage demand.
Manufacturing advances are also being driven by process automation and roll-to-roll electrode fabrication, which enable high-throughput, consistent quality, and reduced labor costs. Eaton, a global supplier of ultracapacitor modules, has integrated automated assembly lines to streamline production and improve scalability. Meanwhile, Panasonic continues to refine its electrode coating and cell assembly techniques, focusing on reducing material waste and improving yield.
Material cost reduction is further supported by the development of alternative electrolytes and binders that are less expensive and more environmentally friendly. For example, water-based electrolytes are being explored to replace organic solvents, reducing both cost and environmental impact. Companies are also working with suppliers to secure reliable sources of precursor materials, such as coconut shell-based activated carbon, to ensure price stability and supply chain resilience.
Looking ahead, the next few years are expected to bring further cost reductions as economies of scale are realized and new materials—such as hybrid metal oxide-carbon composites—are introduced. Industry collaborations and public-private partnerships are accelerating the commercialization of these innovations. As a result, ultracapacitor modules are projected to become increasingly competitive with lithium-ion batteries in applications requiring rapid charge-discharge cycles and long operational lifetimes, particularly in transportation, grid balancing, and industrial power backup.
Key Industry Players and Strategic Partnerships
The ultracapacitor materials engineering sector in 2025 is characterized by a dynamic landscape of established manufacturers, innovative startups, and strategic collaborations aimed at advancing energy storage performance. Key industry players are investing heavily in research and development to optimize electrode materials, electrolytes, and cell architectures, with a particular focus on sustainability, scalability, and integration with next-generation applications such as electric vehicles (EVs), grid stabilization, and industrial automation.
Among the global leaders, Maxwell Technologies (a subsidiary of Tesla, Inc.) continues to play a pivotal role in the development and commercialization of ultracapacitor modules and materials. The company is known for its proprietary dry electrode technology, which is being further refined for higher energy density and longer cycle life. In parallel, Skeleton Technologies, headquartered in Estonia and Germany, is advancing its patented “curved graphene” material, which has demonstrated significant improvements in power density and efficiency. Skeleton’s partnerships with automotive OEMs and grid operators are expected to accelerate the deployment of ultracapacitor-based solutions in Europe and beyond.
In Asia, Panasonic Corporation and Nichicon Corporation remain at the forefront of ultracapacitor component manufacturing, leveraging their extensive experience in electronic materials and large-scale production. Both companies are actively collaborating with automotive and industrial partners to tailor ultracapacitor modules for hybrid and electric mobility platforms. Meanwhile, Eaton and Siemens are integrating ultracapacitor systems into smart grid and industrial automation projects, often in partnership with materials specialists to co-develop advanced carbon-based electrodes and hybrid capacitor technologies.
Strategic partnerships are a defining feature of the current landscape. For example, Skeleton Technologies has entered into joint development agreements with major automotive suppliers to co-engineer ultracapacitor modules for regenerative braking and power buffering. Similarly, Maxwell Technologies is collaborating with battery manufacturers to explore hybrid energy storage systems that combine the rapid charge-discharge capabilities of ultracapacitors with the high energy density of lithium-ion batteries.
Looking ahead, the next few years are expected to see intensified collaboration between material science innovators, component manufacturers, and end-users. The focus will be on scaling up production of advanced carbon materials (such as graphene and carbon nanotubes), improving electrolyte formulations, and developing cost-effective, environmentally friendly manufacturing processes. These efforts are likely to be supported by public-private initiatives and cross-industry consortia, positioning ultracapacitor materials engineering as a critical enabler of the global energy transition.
Application Trends: Automotive, Grid, and Industrial Sectors
Ultracapacitor materials engineering is rapidly evolving to meet the stringent demands of automotive, grid, and industrial sectors, with 2025 marking a pivotal year for both innovation and commercialization. The automotive industry, in particular, is driving significant advancements in electrode and electrolyte materials to enhance energy density, power delivery, and lifecycle performance. Leading manufacturers such as Maxwell Technologies (a subsidiary of Tesla) and Skeleton Technologies are at the forefront, leveraging proprietary carbon-based materials and hybrid architectures to push the boundaries of ultracapacitor capabilities.
In automotive applications, ultracapacitors are increasingly integrated for start-stop systems, regenerative braking, and power buffering in electric and hybrid vehicles. The focus is on materials such as graphene-enhanced activated carbons and carbon nanotubes, which offer high surface area and conductivity. Skeleton Technologies has commercialized its patented “Curved Graphene” material, claiming significant improvements in power density and cycle life, and is supplying modules for heavy-duty vehicles and rail applications across Europe. Meanwhile, Maxwell Technologies continues to supply ultracapacitor modules for automotive OEMs, with ongoing research into advanced carbon composites and hybrid systems that combine lithium-ion and ultracapacitor technologies.
Grid and industrial sectors are also witnessing a surge in ultracapacitor deployment, particularly for grid stabilization, frequency regulation, and uninterruptible power supply (UPS) systems. Here, the engineering focus is on scalability, safety, and operational longevity. Companies like Skeleton Technologies and Eaton are developing large-scale modules and rack-mounted systems, utilizing advanced electrode formulations and robust packaging to withstand harsh industrial environments. The use of environmentally benign electrolytes and high-purity carbon materials is becoming standard, aligning with global sustainability goals.
Looking ahead to the next few years, the outlook for ultracapacitor materials engineering is shaped by the convergence of nanomaterials research, manufacturing scale-up, and sector-specific requirements. The automotive sector is expected to see further integration of hybrid energy storage systems, with ultracapacitors complementing batteries for peak power and fast charging. In grid and industrial domains, modular ultracapacitor banks are anticipated to play a critical role in renewable energy integration and power quality management. As material innovations continue to improve energy and power densities, the adoption curve across these sectors is set to accelerate, with industry leaders like Skeleton Technologies, Maxwell Technologies, and Eaton driving the transition from niche to mainstream applications.
Regulatory Landscape and Industry Standards (ieee.org, sae.org)
The regulatory landscape and industry standards for ultracapacitor materials engineering are evolving rapidly as the technology matures and finds broader adoption in automotive, grid, and industrial applications. In 2025, the focus is on harmonizing safety, performance, and environmental standards to support the integration of advanced ultracapacitor materials—such as graphene, carbon nanotubes, and hybrid composites—into commercial products.
Key industry bodies, including the IEEE and the SAE International, are at the forefront of developing and updating standards that address the unique characteristics of ultracapacitor materials. The IEEE has established the IEEE 1679.1 standard, which provides guidelines for the characterization and performance testing of electric double-layer capacitors (EDLCs) and hybrid capacitors. This standard is being revised to reflect advances in electrode materials, particularly the use of nanostructured carbons and metal oxides, which offer higher energy densities and improved cycle life.
Similarly, SAE International is working on standards that address the integration of ultracapacitors in automotive systems, focusing on safety, reliability, and interoperability. The SAE J3078 standard, for example, outlines test procedures for ultracapacitor modules used in vehicles, with recent updates incorporating requirements for new material classes and thermal management strategies. These standards are critical as automakers and suppliers, such as Maxwell Technologies (a subsidiary of Tesla, Inc.), Skeleton Technologies, and Eaton, accelerate the deployment of ultracapacitor-based energy storage systems.
Environmental and safety regulations are also shaping material choices. The European Union’s REACH regulation and the Restriction of Hazardous Substances (RoHS) directive are influencing the selection of binders, electrolytes, and conductive additives, pushing manufacturers toward greener chemistries and recyclable materials. Companies are responding by investing in sustainable sourcing and end-of-life recycling programs, as seen in initiatives by Skeleton Technologies and Eaton.
Looking ahead, the next few years will likely see the introduction of new international standards that address the lifecycle assessment of ultracapacitor materials, including carbon footprint and recyclability metrics. Collaboration between industry, academia, and regulatory bodies will be essential to ensure that standards keep pace with rapid material innovations, supporting safe and sustainable growth of the ultracapacitor sector.
Market Forecast: Growth Projections and Regional Analysis (2025–2030)
The ultracapacitor materials engineering market is poised for robust growth between 2025 and 2030, driven by accelerating demand for high-performance energy storage in automotive, grid, and industrial applications. The sector’s expansion is underpinned by ongoing advancements in electrode materials—particularly activated carbon, graphene, and hybrid composites—enabling higher energy densities and improved cycle life. As of 2025, leading manufacturers are scaling up production capacities and investing in R&D to address both performance and cost challenges.
Regionally, Asia-Pacific is expected to maintain its dominance, propelled by the presence of major ultracapacitor producers and a strong electric vehicle (EV) supply chain. Companies such as Maxwell Technologies (a subsidiary of Tesla, Inc.), Skeleton Technologies, and Panasonic Corporation are actively expanding their ultracapacitor materials portfolios, with a focus on next-generation carbon nanomaterials and hybrid electrodes. China, in particular, is investing heavily in domestic production of activated carbon and graphene-based materials, aiming to reduce reliance on imports and secure supply for its rapidly growing EV and renewable energy sectors.
In Europe, the push for decarbonization and grid modernization is fostering demand for advanced ultracapacitor modules. Skeleton Technologies, headquartered in Estonia and Germany, is scaling up its production of curved graphene-based ultracapacitors, targeting automotive OEMs and grid operators. The company’s investments in proprietary materials engineering are expected to yield significant performance gains, with projected energy density improvements of up to 60% by 2030.
North America is witnessing increased activity from both established players and startups. Maxwell Technologies continues to innovate in electrode formulation, while Eaton Corporation is integrating ultracapacitor modules into power quality and backup systems. The U.S. government’s emphasis on domestic supply chains and advanced manufacturing is likely to spur further investment in ultracapacitor materials R&D.
Looking ahead, the global ultracapacitor materials engineering market is forecast to achieve double-digit annual growth rates through 2030, with total market value potentially surpassing several billion USD by the end of the decade. Key growth drivers include the electrification of transport, grid resilience initiatives, and the proliferation of renewable energy. However, the market’s trajectory will depend on continued breakthroughs in materials science—particularly in scalable, cost-effective production of high-performance carbon nanomaterials and hybrid electrodes.
Future Outlook: R&D Pipelines and Disruptive Technologies
The landscape of ultracapacitor materials engineering is poised for significant transformation in 2025 and the coming years, driven by intensive R&D pipelines and the pursuit of disruptive technologies. The sector’s focus is on overcoming the traditional trade-offs between energy density, power density, and cycle life, with a particular emphasis on advanced materials and hybrid architectures.
A central trend is the accelerated development of next-generation electrode materials. Companies such as Maxwell Technologies (a subsidiary of Tesla) are investing in novel carbon-based materials, including graphene and carbon nanotubes, to enhance surface area and conductivity. These materials promise to push energy densities beyond the current commercial range of 5-10 Wh/kg, targeting values closer to 20 Wh/kg while maintaining rapid charge/discharge capabilities. Similarly, Skeleton Technologies is advancing its patented “curved graphene” technology, which has demonstrated significant improvements in both energy and power density, and is being integrated into pilot production lines for automotive and grid applications.
Hybrid ultracapacitors, which combine the fast charge/discharge of capacitors with the higher energy storage of batteries, are another focal point. Eaton and Siemens are both exploring hybrid systems that utilize asymmetric electrode configurations—pairing activated carbon with metal oxides or conducting polymers—to bridge the gap between supercapacitors and lithium-ion batteries. These efforts are expected to yield commercial products with improved energy densities and longer operational lifespans by 2026-2027.
Electrolyte innovation is also a key area of R&D. The shift towards environmentally benign, high-voltage electrolytes is being pursued by multiple industry players. CAP-XX is developing aqueous and ionic liquid-based electrolytes that enable higher operating voltages and improved safety profiles, which are critical for applications in electric vehicles and renewable energy storage.
Looking ahead, the integration of AI-driven materials discovery platforms is expected to accelerate the identification and optimization of novel ultracapacitor materials. Companies with strong R&D capabilities and vertically integrated manufacturing, such as Maxwell Technologies and Skeleton Technologies, are well-positioned to capitalize on these advances. The next few years will likely see the commercialization of ultracapacitors with substantially higher energy densities, broader temperature ranges, and enhanced safety, opening new markets in transportation, grid stabilization, and consumer electronics.